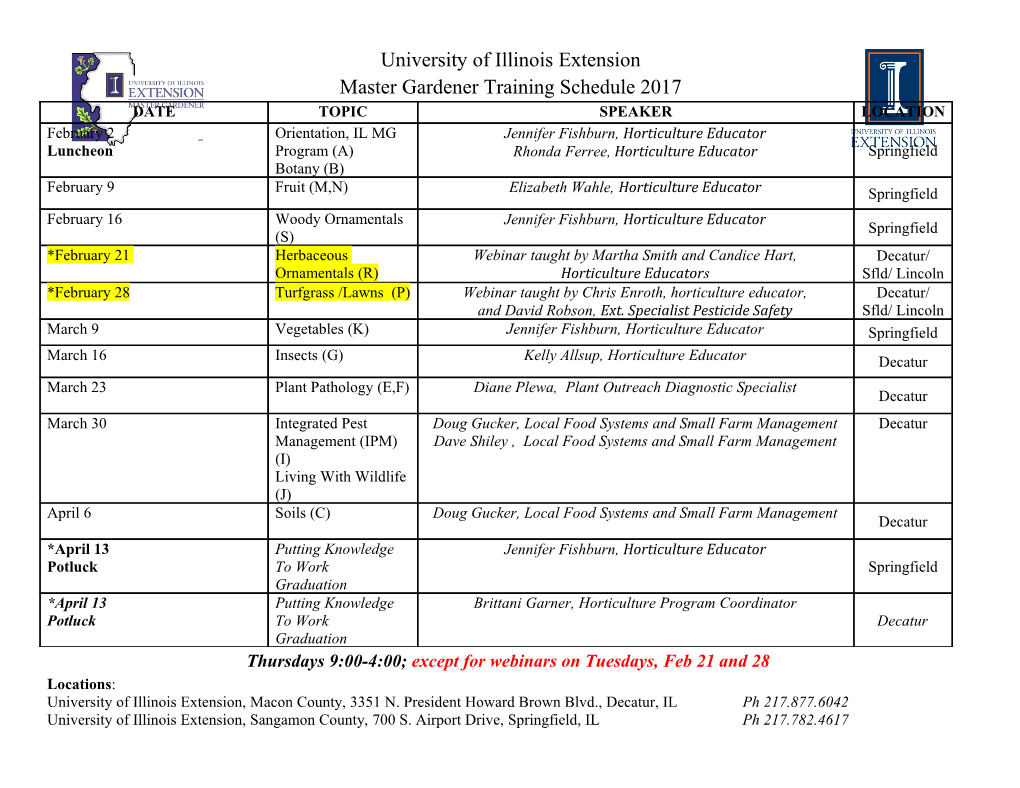
Preprints (www.preprints.org) | NOT PEER-REVIEWED | Posted: 13 June 2017 doi:10.20944/preprints201706.0059.v1 Peer-reviewed version available at J. Clin. Med. 2017, 6, 64; doi:10.3390/jcm6070064 Pathology of Adult and Paediatric Mitochondrial Myopathies Rahul Phadke1,2 1 UCL Institute of Neurology, Division of Neuropathology, National Hospital for Neurology and Neurosurgery, UCLH NHS Foundation Trust, London WC1N 3BG, UK 2 Dubowitz Neuromuscular Centre, Great Ormond Street Hospital for Children NHS Foundation Trust, London WC1N 3JH, UK Correspondence: [email protected]; Tel.: +44-020-344-84393 Abstract Mitochondria are dynamic organelles ubiquitously present in nucleated eukaryotic cells, subserving multiple metabolic functions, including cellular ATP generation by oxidative phosphorylation (OXPHOS). The OXPHOS machinery comprises five transmembrane respiratory chain enzyme complexes (RC). Defective OXPHOS gives rise to mitochondrial diseases (mtD). The incredible phenotypic and genetic diversity of mtD can be attributed at least in part to the RC dual genetic control (nuclear DNA [nDNA] and mitochondrial DNA [mtDNA]) and the complex interaction between the two genomes. Despite the increasing use of next-generation-sequencing (NGS) and various -omics platforms in unraveling novel mtD genes and pathomechanisms, current clinical practice for investigating mtD essentially involves a multipronged approach including clinical assessment, metabolic screening, imaging, pathological, biochemical and functional testing to guide molecular genetic analysis. This review addresses the broad muscle pathology landscape including genotype-phenotype correlations in adult and paediatric mtD, the role of immunodiagnostics in understanding some of the pathomechanisms underpinning the canonical features of mtD, and recent diagnostic advances in the field. Keywords: mitochondrial; muscle biopsy; ragged red; COX-negative; subsarcolemmal; immunohistochemistry 1. Introduction The diagnosis of mtD is challenging due to the incredible phenotypic and genetic diversity associated with these diseases. This partly stems from the dual genetic control (nDNA and mtDNA) of the RC, the complexity of intergenomic signaling and its functional consequences. mtD can be inherited in an autosomal dominant, autosomal recessive, X-linked or mitochondrial (i.e. maternal) fashion. The circular mtDNA encodes 13 RC subunits, 22 mitochondrial tRNAs and 2 ribosomal RNAs. Additionally, the mitoproteome requires over 1300 nuclear encoded proteins to produce, assemble and support the five multimeric OXPHOS RC (I-V), and ancillary mitochondrial processes1-3. Tissues and organs affected in mtD are often those with high-energy requirements. Clinical symptoms can manifest at any age, and can affect a single organ or be multisystemic4. Typically the more severe phenotypes present early, and milder phenotypes present later in life5. There are classic clinical syndromes with stereotypic features such as © 2017 by the author(s). Distributed under a Creative Commons CC BY license. Preprints (www.preprints.org) | NOT PEER-REVIEWED | Posted: 13 June 2017 doi:10.20944/preprints201706.0059.v1 Peer-reviewed version available at J. Clin. Med. 2017, 6, 64; doi:10.3390/jcm6070064 Leigh syndrome (subacute necrotising encephalomyopathy), MELAS (mitochondrial myopathy, encephalopathy, lactic acidosis and stroke-like episodes) and Alpers disease (epilepsy and liver failure). However, many patients display non-specific features of developmental delay or regression, further hindering accurate diagnosis6. The onset of symptoms, phenotypic variability, and variable penetrance of mtD are influenced by the peculiarities of mitochondrial genetics including the threshold effect, mitotic segregation, clonal expansion and a genetic bottleneck, as well as the nuclear genome background in which it coexists or by environmental and epigenetic factors6,7. 2. Laboratory investigations and the rationale for muscle biopsy Given the complexity of mtD phenotypes and genetics, securing a diagnosis frequently requires extensive non-invasive and invasive tests including imaging, neurophysiology, metabolic and biochemical studies, muscle pathology and functional testing, followed by definitive molecular genetic confirmation. Resting and exercise induced increase in blood lactate is a useful albeit non-specific screening tool for mtD, but can be normal or minimally elevated as in mitochondrial polymerase gamma (POLG1) associated diseases, Leber Hereditary Optic Neuropathy (LHON), Leigh disease, Kearns-Sayre syndrome and Complex I deficiency8. The blood lactate/pyruvate ratio may increase in inborn errors of the mitochondrial respiratory chain9. Spurious elevation of plasma lactate and/or pyruvate may occur from poor collection or handling technique, secondary mitochondrial dysfunction in a range of systemic and metabolic diseases, and in nutritional thiamine deficiency. Blood and/or CSF pyruvate levels may increase in defects of pyruvate metabolism. Similarly, CSF lactate and/or pyruvate levels may increase without blood elevation in mtD with predominant CNS manifestations10. Elevated plasma/CSF amino acids, urine organic acids, and plasma acylcarnitines all suggest underlying mitochondrial dysfunction, however, normal levels do not exclude mtD11, 12. CK values are normal or mildly elevated, unless measured in the setting of rhabdomyolysis13. Neurophysiology may show non-specific signs of a myopathy, or a neuropathy when present, but may be normal. There is no single ‘gold standard’ laboratory test for diagnosing mtD. The screening tests described above broadly confirm presence of dysfunction in various organ systems and help to increase or decrease the clinical suspicion of mtD. More invasive testing is necessary to establish direct morphological, biochemical and molecular genetic evidence of mitochondrial dysfunction5. In principle, the relevant tissue to investigate is one that clinically expresses disease. Skeletal muscle remains the tissue of choice, and is frequently sampled in part due to the relative safety and ease with which tissue samples can be obtained. It can provide valuable diagnostic information in many cases, even without clinically overt myopathic involvement14-16. Skeletal muscle is a post- mitotic terminally differentiated tissue with only limited regenerative capacity via satellite cell transformation. This terminal differentiation results in a fairly stable lifelong relationship between the mutant and wild-type mtDNA ratio (heteroplasmy) in contrast to nucleated blood cells in which this ratio can decrease due to selection pressure, thereby obscuring evidence of mitochondrial dysfunction16. Skeletal muscle mitochondria are abundant in subsarcolemmal Preprints (www.preprints.org) | NOT PEER-REVIEWED | Posted: 13 June 2017 doi:10.20944/preprints201706.0059.v1 Peer-reviewed version available at J. Clin. Med. 2017, 6, 64; doi:10.3390/jcm6070064 and intermyofibrillar locations and larger than in most other tissues. Pathological assessment of ‘non-muscle’ components in biopsies including blood vessels and nerves can provide evidence of multi-organ dysfunction15. Biochemical testing of respiratory chain enzyme dysfunction typically involves determination of individual or paired respiratory chain enzyme complex activities in mitochondrial fractions or tissue homogenates prepared from fresh or frozen muscle tissue. Biochemical assays have low inter-laboratory reproducibility and a systematic program to share samples and standardise methodologies across diagnostic laboratories hasn’t been implemented. Other confounding factors include masking of a RC defect in tissue homogenates due to low-level heteroplasmy and a physiological compensatory mitochondrial proliferative response6. In this context, parallel histological assessment of skeletal muscle can uniquely provide histochemical evidence of RC defect at the single cell level. Simultaneously the biopsy can be assessed for a number of conditions in the clinical differential diagnosis that can mimic a mitochondrial myopathy or induce secondary mitochondrial dysfunction. This includes fatty acid oxidation defects, glycogen storage disorders, endocrine, congenital and inflammatory myopathies and muscular dystrophies. The reliability of detecting morphological and histochemical abnormalities in skeletal muscle in mtD has led to their inclusion as major and minor criteria in several classification schemes for diagnosing mtD in adults and children17-20. It is standard practice to perform a skin biopsy in parallel to a muscle biopsy primarily for establishing fibroblast cultures. While it is less invasive, it is not uncommon for patients with OXPHOS defects in skeletal muscle to have normal RC activities in fibroblasts21, 22. This in part due to altered heteroplasmy and high tissue regeneration rate of fibroblasts compared to skeletal muscle21. It is equally important to recognise the limitations of muscle biopsy analysis in investigating mtD. RC deficiencies are usually tissue specific, particularly if sporadic and somatic, further influenced by the type of mutation and the peculiarities of mitochondrial genetics. Therefore even muscle samples with proven mtD mutations/phenotypes may not show pathological and/or biochemical evidence of mitochondrial dysfunction13. mtDNA copy number analysis in muscle tissue by real time qPCR normalised to age-matched controls gives an indication of depletion or amplification of mtDNA content. mtDNA depletion can point towards
Details
-
File Typepdf
-
Upload Time-
-
Content LanguagesEnglish
-
Upload UserAnonymous/Not logged-in
-
File Pages30 Page
-
File Size-