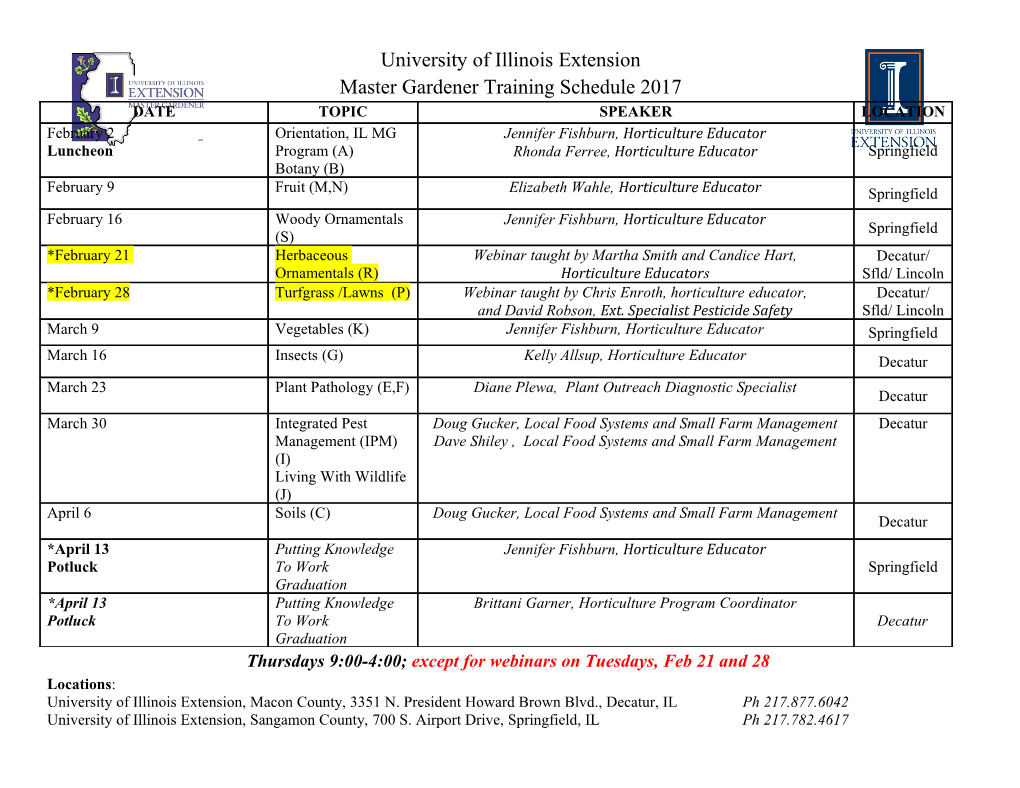
CONTENTS SUMMARY AND CONCLUSIONS PAPERS ON CHROMOSPHERIC LINES IN RED DWARF FLARE STARS I. AD LEONIS AND GX ANDROMEDAE B. R. Pettersen and L. A. Coleman, 1981, Ap.J. 251, 571. II. EV LACERTAE, EQ PEGASI A, AND V1054 OPHIUCHI B. R. Pettersen, D. S. Evans, and L. A. Coleman, 1984, Ap.J. 282, 214. III. AU MICROSCOPII AND YY GEMINORUM B. R. Pettersen, 1986, Astr. Ap., in press. IV. V1005 ORIONIS AND DK LEONIS B. R. Pettersen, 1986, Astr. Ap., submitted. V. EQ VIRGINIS AND BY DRACONIS B. R. Pettersen, 1986, Astr. Ap., submitted. PAPERS ON FLARE ACTIVITY VI. THE FLARE ACTIVITY OF AD LEONIS B. R. Pettersen, L. A. Coleman, and D. S. Evans, 1984, Ap.J.suppl. 5£, 275. VII. DISCOVERY OF FLARE ACTIVITY ON THE VERY LOW LUMINOSITY RED DWARF G 51-15 B. R. Pettersen, 1981, Astr. Ap. 95, 135. VIII. THE FLARE ACTIVITY OF V780 TAU B. R. Pettersen, 1983, Astr. Ap. 120, 192. IX. DISCOVERY OF FLARE ACTIVITY ON THE LOW LUMINOSITY RED DWARF SYSTEM G 9-38 AB B. R. Pettersen, 1985, Astr. Ap. 148, 151. Den som påstår seg ferdig utlært, - han er ikke utlært, men ferdig. 1 ATMOSPHERIC ACTIVITY IN RED DWARF STARS SUMMARY AND CONCLUSIONS Active and inactive stars of similar mass and luminosity have similar physical conditions in their photospheres/ outside of magnetically disturbed regions. Such field structures give rise to stellar activity, which manifests itself at all heights of the atmosphere. Observations of uneven distributions of flux across the stellar disk have led to the discovery of photospheric starspots, chromospheric plage areas, and coronal holes. Localized transient behavior has been identified in both thermal and non-thermal sources, such as flares, shock waves and particle acceleration. The common element to all active regions is the presence of strong magnetic field structures connecting the violently turbulent deep layers in the convection zones of stars with the tenuous outer atmospheres. Transport and dissipation of energy into the chromospheric and coronal regions are still much debated topics. PROPERTIES OF STELLAR FLARES A stellar flare is observed as an increase in radiation from all levels of the stellar atmosphere. In addition to a very strong optical and ultraviolet continuum, one detects X-rays and radio waves from the corona and emission lines from the transition region and the chromosphere. Continuum dominates over radiation losses in emission lines during the phase of maximum radiation. In contrast, the "white light" continuum is rarely seen in solar flares. The optical flare event is characterized by a rapid increase in brightness, often followed by an almost equally rapid decay, but the main part of the duration of a flare is taken up by a slow decay in brightness till the pre- flare level is again reached. Many parameters of the flare light curve vary by two orders of magnitude on the same star, such as timescales of rise and decay, and the flare amplitude. The total energy emitted in optical light during a flare varies by much more. On some stars we detect flares as small as IO*7 ergs. The largest events have emitted in excess of 1035 ergs. The flux at maximum spans more than four orders of magnitude. The frequency distributions of flare energy ,^jrshberg 1972) and flux at flare maximum (Kunkel 1973) demonstrate that large flares, both in terms of energy and amplitude, are much less common than small flares. As the technical ability to detect smaller flares has improved over the years, it has been demonstrated that the distributions continue to hold. There is no indication yet of "a smallest possible flare", below which no flare event is recorded. Numerical values for the slope of the cumulative frequency distribution of flare energy vary from star to star. It is not clear if this is a property of the star, or if it is due to observational effects. In Fig. 1, which compiles distributions for a number of stars, it appears a collective property that all stars have similar slope values. For solar neighbourhood stars the values range from 0.4 to 1.0, and show some tendency of correlation with stellar luminosity. T 1 i i r log Ey (ergs) 34 32 30 28 26 i • I 1 1 l 1 i -6 -4-2 0 +2 log N/T (hour"') Fig. 1. - Cumulative frequency distributions of flare energy for a number of stars. Photometric observations of flares have revealed a number of statistical properties of flare activity on stars. Some are due to observational selection effects connected to the contrast between flare light and the photospheric brightness. Energetic flares are seen mostly on intrinsically bright stars, while low energy flares are seen on faint stars. Since small flares occur more frequently than large flares we observe that intrinsically faint stars flare more often than bright stars. The relative contribution of flare light to the total flux from a star is larger in faint stars than in bright ones. The low energy cut-off as a function of stellar luminosity is apparent in Fig. 1. Whether low luminosity stars are capable of producing very energetic flares cannot be decided from existing observations. Such stars must be observed with large telescopes where observing time is precious, so only a few hours of monitoring have been accumulated on most of these objects. The bright stars can be observed with small telescopes and require vast amounts of observing time for each detected flare. Since the sampling times are so different, the conditions favour detection of very energetic flares in bright stars. It may be indicative, however, that 95 hours of U-filter monitoring on YY Gem (Mv°9) revealed flares as large as 1034 ergs, while 93 hours on W Cet (^=15) resulted in none larger than 5 1031 ergs. The opposite question, whether all stars produce low energy flares, cannot be investigated with optical methods due to contrast effects. Extended X-ray monitoring (and possibly in UV) would provide us with data for addressing this problem. Il When the observations are calibrated to scales of absolute physical units, the stellar luminosity in the U-filter, Ly, and the time averaged flare energy, Lj(U)( are both in ergs s"1. Total absence of low energy flares in bright stars leads to undesestiroates of their Lf(V)-values. Weaker flares can be detected in faint stars so there the sampling is more complete over the range of produced energies. Fig. 2 shows that the time averaged flare energy emitted by a star increases with increasing ste.llar luminosity. Discarding stars of unusually low activity, often with no emission lines in their spectra, we find that 2/3 Lf (U) a L„ (1) 32 1 —i 1 1— —P- log Ly • • (ergs s" ) • • / 30 m/ - • • Y - •J 28 . fa • 26 - 3 2 - Lf ' 24 —i _i _i_ ,.J i 24 26 28 30 -1, log Lf(U) (ergs s ') Fig. 2. " Time averaged flare energy for a number of stars. The observed flare frequencies are also affected by various selection effects. We have attempted to avoid these by utilizing the observed distributions of flare energy to determine the flare frequency (in flares per hour) for flares larger than a fixed value, i.e. N/T(E>EQ ergs). Since a rare large flare in a low luminosity star may be barely detectable in a bright star, we have obo£?n 29 30 Eo-10 and 10 ergs. This choice minimizes the range of extrapolation. Discarding stars of unusually low activity, we find that 1/2 (2) This relationship, which holds for five orders of magnitude in stellar luminosity, is shown in Pig. 3. Flares larger than a given energy are more frequent on bright stars than on low luminosity stars. Since this holds for several choices of energy, we suggest that in general the frequency of flares of a given energy is larger on bright stars than on faint stars. 4 it it. 09 lu - ergs s" ) • / • / • • / 30 • 30 • / . • • / • •f/• 28 28 • • • / 26 26 - / , , 24 1 b 1 1 24 —u 1 ,i i -2 0 +2 -2 0 +2 29 30 loq N/T(Eu>10 erqs) log N/T(Eu>10 ergs) (flares per hour) (flares per hour) Fig. 3. - Flare frequencies for stars of different luminosity. In summary, the results of Figs. 2 and 3 suggest that bright stars are more flare active than low luminosity stars. Theie are also indications of different activity levels for stars of similar luminosity, which may indicate that other parameters also play a role (e.g. age, rotation). Studies of flare light curves have revealed the existence of a relationship between the average flare decay rate and the luminosity of the star. An updated version of this relationship is shown in Fig. 4. It has a break near MyslO, which coincides with a similar break in the mass-luminosity relation (Pettersen 1983). For the entire luminosity range 7<MV<17 (magnitudes) one thus obtains . i 0 t. « — a <r (3) 5 °- g2 where tg.5 is the flare decay timescale, g is the surface gravity of the star, and h is the pressure (gravitational) scale height in its photosphere. For low luminosity stars (My=15) we detect flares as weak as 1027 ergs. Only rarely do we see sinole maximum flares with energies larger than 1031 ergs. The average flare decay timescale is therefore determined mainly by weak flares. Brighter stars like EV Lac and AD Leo (My»ll) have more intense photospheric radiation so the flare contrast is lower. We detect flares between 10^9 and 1Q33 ergB for these stars.
Details
-
File Typepdf
-
Upload Time-
-
Content LanguagesEnglish
-
Upload UserAnonymous/Not logged-in
-
File Pages164 Page
-
File Size-