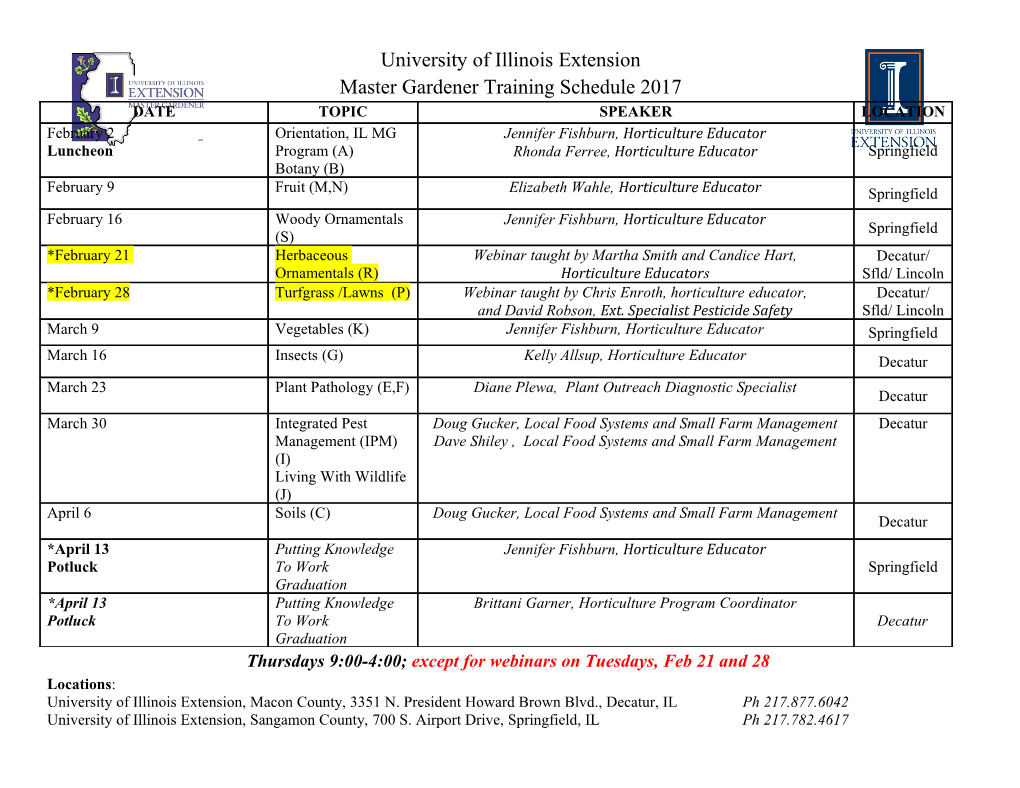
62 Journal of Food Protection, Vol. 55, No. I, Pages 62-70 (January 1992) Copyright©, International Association of Milk, Food and Environmental Sanitarians Bacterial Bioluminescence: Applications in Food Microbiology J. M. BAKER', M. W. GRIFFITHS', and D. L. COLLINS-THOMPSON2 Departments of !Food Science and ^'Environmental Biology, University of Guelph, Guelph, Ontario NIG 2W1, Canada Downloaded from http://meridian.allenpress.com/jfp/article-pdf/55/1/62/2303097/0362-028x-55_1_62.pdf by guest on 30 September 2021 (Received for publication June 10, 1991) ABSTRACT a biochemical and genetic level. The applications of bacte­ rial bioluminescence will also be presented, with an empha­ Many marine microorganisms (Vibrio, Photobacterium) are sis on the applications in food microbiology. capable of emitting light, that is, they are bioluminescent. The light-yielding reaction is catalyzed by a luciferase, and it involves BIOCHEMISTRY OF THE the oxidation of reduced riboflavin phosphate and a long-chain BIOLUMINESCENT REACTION aldehyde in the presence of oxygen to produce a blue green light. The genes responsible for the luciferase production, (lux A and lux B), aldehyde synthesis (lux C, D, and E), and regulation of The bioluminescent reaction, catalyzed by the enzyme luminescence (lux I and lux R) have all been identified, and recent luciferase, involves the oxidation of a long-chain aldehyde research has resulted in the discovery of three new genes (lux F, and reduced riboflavin phosphate (FMNH2) and results in G, and H). The ability to genetically engineer dark microorgan­ the emission of a blue green light. te fc e isms to become light emitting by introducing the lux genes into FMNH2 + 02 + RCOH — ' ™ —> them has opened up a wide range of applications of biolumines­ FMN + RCOOH + H20 -(-light (490 nm) cence. Assays using bacterial bioluminescence for the detection The primary source of energy for the light is supplied by and enumeration of microorganisms are rapid, sensitive, accurate, the conversion of the aldehyde to the corresponding fatty and can be made specific. It is these attributes that are making in acid. Long-chain aldehydes are essential for the lumines­ vivo bioluminescent assays so attractive to the food industry. cence reaction and the aldehyde, tetradecanal, appears to be the natural substrate for the luminescence reaction (25). The reaction is highly specific for FMNFL, which is formed by the reaction: Bioluminescent bacteria (those capable of emitting FMN light) are classified into four major genera: Vibrio, NAD(P) H + FMN — °»^^^— > FMNH2 Photobacterium, Alteromonas, and Xenorhabdus. They are Thus, the luciferase reaction may be driven by coupling it classified as motile, Gram-negative rods, and function as to any system that produces FMNH2. Electrons for the facultative anaerobes (25,26). The first three genera are reduction of flavin mononucleotide (FMN) are provided by marine in origin and can be isolated from seawater or the reducing power derived from the electron transport certain luminous ocean species. They can exist free living pathway (75). The mechanism of the reaction leading to in the ocean, as symbionts with various marine fish in their light emission is shown schematically in Fig. 1. FMNH2 digestive tracts or specialized light organs, as saprophytes plus the luciferase results in luciferase-bound FMNH2. on dead fish, and as parasites in Crustacea and insects (26). Luciferase-bound FMNH2 reacts with oxygen to form the The marine organisms coexisting with the luminescent 4a-peroxyflavin intermediate. The intermediate reacts with bacteria can use the luminescence for a variety of purposes a long-chain aldehyde to form an excited species - the such as attraction of prey, communication between species, flavin-4a-hydroxide (72). This compound is highly stable and escape from predators. However, it is not known what and decays slowly resulting in the light emission and the specific benefit these bacteria derive from this energy oxidation of the substrates (26). consuming property, either free living or when associated with higher organisms (77). Detailed biochemical and ge­ THE ENZYME LUCIFERASE netic studies have been carried out on species of Vibrio and Photobacterium. The results of these studies have led to an Luciferase is the enzyme catalyzing the bioluminescent advancement in the understanding of bacterial biolumines­ reaction and it is linked to the respiratory pathway. It is an cence. Researchers are now beginning to discover a wide external flavin mono-oxygenase or mixed function range of applications and uses for bioluminescence. This oxygenase. All luciferases are heterodimers (77 kDa) con­ review will cover the luminescence reaction in bacteria at sisting of two nonidentical subunits a (40 kDa) and [3 (37 JOURNAL OF FOOD PROTECTION, VOL. 55, JANUARY 1992 BACTERIAL LUMINESCENCE 63 hv tern. There are seven genes and two operons required for NAD(P)H + H A NADPH + HT the complete Lux phenotype. Five of the seven genes are FMN RCOOH "-"•,- ATP structural, coding for the luciferase and the fatty acid reductase. The other two genes are regulatory, responsible FATTY ACID 0X1DO- LUCIFERASE for producing an autoinducer and a receptor. The two REDUCTASE REDUCTASE operons are transcribed in opposite directions. The right | I L AMP+ ppi operon carries genes for the autoinducer, luciferase, and the NAD(P) .J^ FMNH„ RCHO V V NADP"+1 fatty acid reductase. The left operon carries the gene for the receptor protein. Fig. 2 shows the organization of the Vibrio fischeri lux genes. Figure 1. Schematic representation of the biochemical pathways involved in bacterial bioluminescence. [From Engebrecht el al, 1983 (11)]. Operon Downloaded from http://meridian.allenpress.com/jfp/article-pdf/55/1/62/2303097/0362-028x-55_1_62.pdf by guest on 30 September 2021 kDa) (25,26). The active site is located primarily on the a subunit, but the p subunit is still essential for the light- emitting reaction and may contribute to the structure of the active centre. Neither the a or p subunit alone exhibits Regulation Aldehyde Luciferase Aldehyde -J I I l_ luciferase activity, but both preparations regain activity 0 1 2 3 4 5 6 7 8 9 when combined with the second subunit - leading to the kbp conclusion that an individual subunit does not appear to possess an active centre. Studies indicate that the aldehyde Figure 2. Organization of the Vibrio fischeri lux genes. [Modified from binding site is, like the FMNH2 binding site, at or near the Hastings el al, 1985 (13)]. interface of the luciferase a and p subunits (75). Luciferase genes lux A and lux B ALDEHYDE BIOSYNTHESIS The a subunit is coded for by lux A and lux B codes for the p subunit. Both genes are approximately 1 kbp in Aldehydes are essential in the bioluminescent reaction length and are located immediately adjacent to one another and luminescent cells have a mechanism of synthesizing and are transcribed in the same direction. Aldehyde depen­ aldehydes from fatty acids. A multienzyme fatty acid re­ dent luminescence requires only a 2.5 kbp DNA fragment ductase complex that produces aldehydes has been purified with the lux A and B genes under a suitable promoter. Only and characterized from Photobacterium phosphoreum (25). the luciferase genes are necessary for luminescence (25). It The net reaction: is now known that lux A and lux B arose by gene duplica­ R-COOH + ATP + NADPH tion (6), and there is about 30% identity in amino acid —-> R-CHO + AMP +PPi +NADP sequence between any luciferase a and p subunits regard­ is the reduction of fatty acid to aldehyde with the oxidation less of bacterial species (4,14,38). of NADPH and the cleavage of ATP to AMP and PPi. Maximum activity is obtained with tetradecanoic acid (25). Fatty acid reductase genes lux C, D, and E Two enzyme activities are necessary for the reaction to The genes coding for aldehyde synthesis, lux C, D, and occur. Acyl-protein synthetase is responsible for the ATP E, must also be transformed and expressed in E. coli to dependent activation of the fatty acid, and a reductase obtain light emission without addition of aldehyde. The lux component catalyzes the NADPH-dependent reduction of C gene encodes for the reductase, the lux D gene encodes the activated fatty acid. Activation of the carboxyl groups for the acyl transferase, and the lux E gene encodes for the is catalyzed by the synthetase and results in an acyl-AMP acyl-protein synthetase (25). intermediate being formed. A NADPH linked reduction of the acyl group to aldehyde is effected by the reductase Regulatory genes lux 7 and lux R (25,26). Acyl-transferase is also present in the fatty acid Two regulatory genes, lux I and lux R, involved in the reductase complex and is involved in the generation of fatty genetic control of luminescence, have been identified in V. acids. Current data suggest that acyl-transferases are in­ fischeri MJl (11,12). The genes lux F, lux G, and lux H volved in diverting fatty acids into the luminescence system have recently been identified (36,37). Lux F codes for a from the lipid biosynthesis pathway. flavoprotein of about 24 kDa with no known function at this time. Lux G and lux H are both 25 kDa proteins also THE lux GENES of unknown function. Meighen speculates on some possible functions of these new proteins and also provides an up­ Escherichia colt cells have been transformed with dated series of organizational maps (26). The in-depth cloned genes coding for the luminescence functions and study of luminescent bacteria on a molecular level is expression has resulted in light emission. This has ad­ starting to result in exciting discoveries about these organ­ vanced understanding of the gene organization and regula­ isms and the mechanisms responsible for their unique tion of luminescence in the bacterial bioluminescence sys- characteristic.
Details
-
File Typepdf
-
Upload Time-
-
Content LanguagesEnglish
-
Upload UserAnonymous/Not logged-in
-
File Pages9 Page
-
File Size-