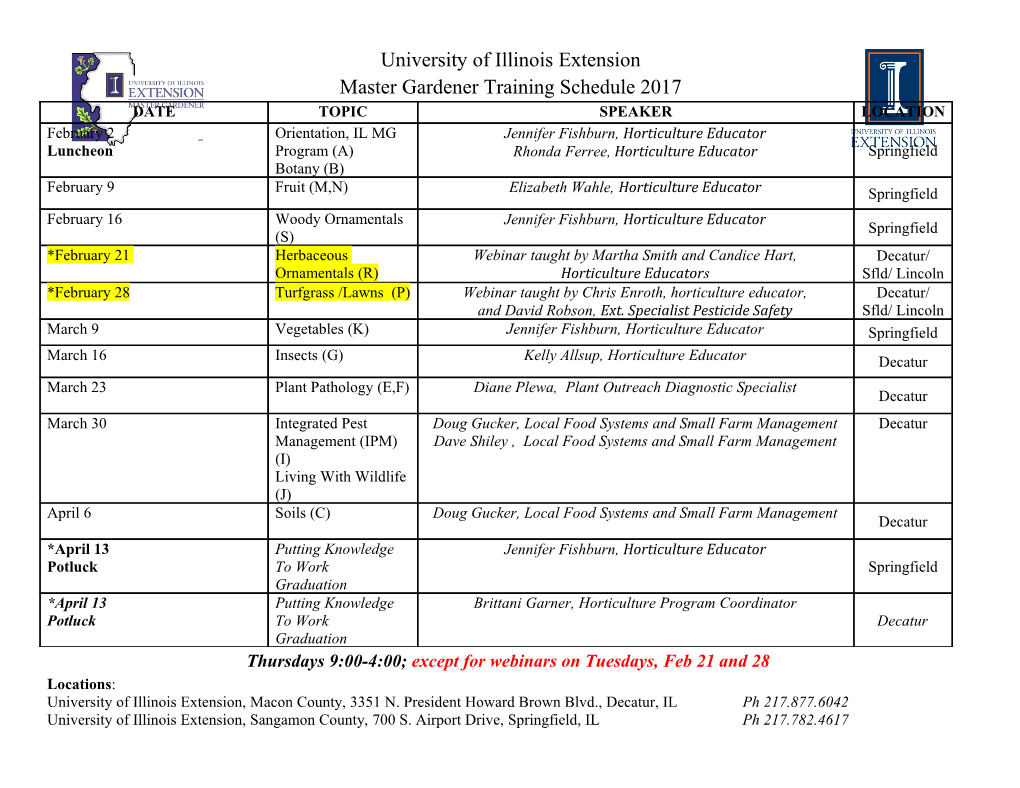
The synthesis of peptides and proteins containing non-natural amino acids David R. W. Hodgson* and John M. Sanderson* Department of Chemistry, South Road, Durham, UK DH1 3LE. E-mail: [email protected]; [email protected]; Fax: 144 (0)191 384 4737; Tel: 144 (0)191 334 2100 Received 16th February 2004 First published as an Advance Article on the web 13th August 2004 Methods for the incorporation of non-natural amino acids into proteins have advanced significantly over recent years and in this tutorial review we aim to give a general overview of the area. These techniques offer the possibility of modulating the structures and functions of proteins and thus permit the generation of novel designed systems for both biocatalytic and mechanistic studies. Four complementary approaches are discussed in detail along with examples of their application. The advantages and disadvantages of each technique are also discussed. Introduction approach relies on the existing functionality of a protein, and through the use of appropriate chemical reagents, chosen The wish to generate designer proteins that rival the properties of amino acid side chains can be modified. All side chains their natural counterparts has been a long sought after goal. The displaying reactive functionality can be modified, and, depend- complexities involved in the de novo design of proteins are ing on the functionality in question and the choice of modifying overwhelming and current computational methods do not permit reagent, highly selective, quantitative modifications can take any great degree of freehand protein design. Therefore many place. Clearly quantitative, site-selective bioconjugations are researchers apply protein engineering techniques to existing the most desirable outcome as these lead to homogeneous protein scaffolds in order to derive new systems that retain the populations of modified products that are suitable for quan- desirable traits of the original system in addition to the newly titative experimental studies and analysis. This section of the designed properties. However standard protein engineering review will discuss some of the most widely used bioconjuga- techniques limit the user to the standard twenty commonly tion systems in order to give the reader a flavour of the types of encountered amino acids. Thus the possibilities in terms of reagents in use and also the end uses of the remolded protein producing modified proteins with new properties or functions products. In addition the advantages and disadvantages of using standard engineering methods are severely limited. To the approach will be discussed. Several other authors have overcome this obstacle, several strategies for the incorporation of reviewed the area comprehensively and the reader is referred to non-natural amino acids into naturally occurring protein and these articles and references therein for a fuller picture of the peptide molecules have been developed. This review will focus on subject.1,2 The reader is also referred to an excellent textbook four strategies that have been widely exploited to date, and are that gives detailed experimental protocols for a wide variety of still undergoing continual advancement and optimisation. bioconjugation experiments.3 Bioconjugation Cysteine modification Bioconjugation is the simplest and longest standing method for The most widely used bioconjugation strategy exploits the the introduction of non-natural amino acids into proteins. The latent nucleophilicity of the thiol side chain of cysteine. Dr David Hodgson graduated from the University of Cambridge Dr John Sanderson obtained his PhD from the University in 1995 with a B.A. in Natural Sciences. He then undertook of Leeds in 1996. He worked as a postdoctoral researcher a PhD on small molecule enzyme at the University of Sheffield model systems, again at Cam- for one year, and then in the bridge, with Professor Tony group of Professor J.-M. Lehn Kirby. In 1999 he moved to at the Colle`ge de France, Paris, the University of Toronto to until September 1999. Upon work on the chemical modifica- returning to the UK, he took tion of subtilisins with Professor up a lectureship at the University J. Bryan Jones. The summer of of Sussex. Since 2001 he has 2000 saw a move to SUNY at been a lecturer in the Department Buffalo to work on ribozyme of Chemistry at the University selection with Hiroaki Suga of Durham, where his research with support from a Royal interests include the design Society-Fulbright post-doctoral and synthesis of peptides fellowship. In January 2003 he and the study of peptide–lipid took up a lectureship at the interactions. David Hodgson University of Durham. John Sanderson DOI: 10.1039/b312953p 422 Chem. Soc. Rev., 2004, 33,422–430 This journal is ß The Royal Society of Chemistry 2004 Through the use of appropriate electrophiles thiol groups can contains Glu78 and Glu172, which lie y5.5 A˚ apart and be rapidly, selectively and quantitatively modified. Cysteine function as the active site nucleophile and the general acid modifications fall into two major categories: alkylation and catalyst respectively. In previous work a Glu78Asp mutant, mixed disulfide formation. Within these categories further sub- where the carboxylate–carboxylate distance is larger than in the divisions can be made according to the choice of modifying wild-type enzyme, was studied, but was found to have little reagent employed. catalytic activity. Therefore a new study centred on reducing the carboxylate–carboxylate distance by introducing a length- Cysteine alkylation ened carboxylic acid analogue of Glu78 was undertaken. Unfortunately, unlike the case for the chain-shortened Probably the most widely used approach is alkylation using Glu78Asp mutant, the standard twenty amino acid repertoire a-halo carbonyl compounds in order to generate thioether does not contain a lengthened-chain analogue of glutamic products (Scheme 1). Under mildly basic (pH 9–10) conditions acid; therefore a combined site-directed mutagenesis (SDM)– chemical modification strategy was employed in order to overcome this limitation. First a cysteine mutant Glu78Cys of the xylanase was produced through SDM. The use of SDM and in vivo expression systems allows relatively large quantities of the mutant protein to be produced in a conventional manner. However, in vivo expression systems limit the user to the twenty naturally occurring amino acids, therefore if the wild-type protein already contains a cysteine residue multiple labelling Scheme 1 Thiol alkylation using a-halo carbonyl compounds. can occur. Fortunately, wild-type B. circulans xylanase does not contain any cysteine residues, thus the Glu78Cys mutation the acidic thiol group can be partially deprotonated and reveal introduces a unique thiol functional group into the xylanase the more nucleophilic thiolate anion. The soft character of this which can then be modified in a highly selective manner. nucleophile complements the electrophilic characteristics of Iodoacetic acid was chosen as the electrophilic modifying a-halo carbonyl compounds and thioether formation occurs reagent with the resultant protein displaying an added rapidly between these components. Furthermore a-halo methylene-carboxy group extending from the added cysteine carbonyl compounds show little reactivity towards other pro- residue (Fig. 1). teinogenic functional groups; thus cross-reactivity is limited, and the products of the modification process tend to be highly homogeneous. An illustrative example of this approach was used by Withers in order to gain insight into the mechanisms of glycosyl hydrolases.4 Glycosyl hydrolases usually contain two catalytic active site carboxylate residues, and the relative positioning of these two carboxyl groups is known to be a critical parameter in determining whether the glycosyl hydrolase in question is either an inverting or retaining hydrolase. One carboxylate is deprotonated and acts as a nucleophile in order to trap the forming oxocarbenium ion, whereas the other is protonated and Fig. 1 (a) Wild-type and (b) modified xylanase active sites. acts as a general acid catalyst in order to facilitate the departure of the alkoxy leaving group of the aglycon (Scheme 2). The extent of thiol modification was then confirmed using Ellman’s Reagent5 and electrospray mass spectrometry (ESMS) was used in order to confirm that the expected modification had indeed taken place. Together this information was used to conclude that the modified protein was homogenous and suitable for detailed kinetic analyses. The Glu78Cys–CH2– 2 CO2 mutant protein retained a significant level of activity with kcat/KM values only 16–100-fold lower than the WT enzyme. The authors concluded that the modified active site nucleophile was still able to participate in nucleophilic catalysis whilst only causing moderate perturbations in the active site conformation owing to its increased size compared to the WT Glu residue. The example cited above illustrates the use of an a-halo compound in the production of a modified enzyme for mechanistic studies on its existing glycosyl transferase activity. However bioconjugated proteins have also been employed in order to ascertain structural information upon the active sites of proteins using a variety of thiol-reactive agents including fluorophores and the structural probe Fe-BABE.6 Scheme 2 Mechanism of a retaining glycosyl hydrolase. Disulfide formation Disulfides offer two particular advantages over and above Withers’ team chose to study the effects of perturbing other thiol-selective
Details
-
File Typepdf
-
Upload Time-
-
Content LanguagesEnglish
-
Upload UserAnonymous/Not logged-in
-
File Pages9 Page
-
File Size-