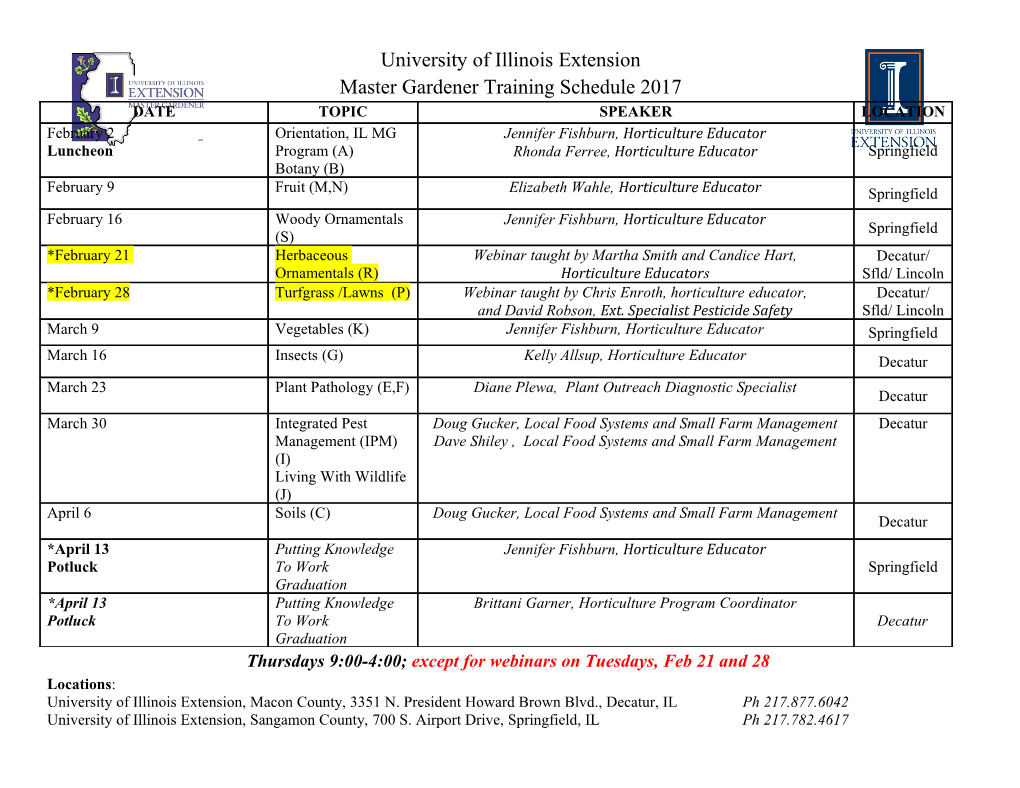
Strong tectonic and weak climatic control of long-term chemical weathering rates Clifford S. Riebe* James W. Kirchner Department of Earth and Planetary Science, University of California, Berkeley, California 94720-4767, USA Darryl E. Granger Department of Earth and Atmospheric Sciences, Purdue University, West Lafayette, Indiana 47907, USA Robert C. Finkel Center for Accelerator Mass Spectrometry, Lawrence Livermore National Laboratory, Livermore, California 94551, USA ABSTRACT The relationships among climate, physical erosion, and chemical weathering have remained uncertain, because long-term chemical weathering rates have been dif®cult to measure. Here we show that long-term chemical weathering rates can be measured by combining physical erosion rates, inferred from cosmogenic nuclides, with dissolution loss- es, inferred from the rock-to-soil enrichment of insoluble elements. We used this method to measure chemical weathering rates across 22 mountainous granitic catchments that span a wide range of erosion rates and climates. Chemical weathering rates correlate strongly with physical erosion rates but only weakly with climate, implying that, by reg- ulating erosion rates, tectonic uplift may signi®cantly accelerate chemical weathering rates in granitic landscapes. Keywords: geochemical mass balance, cosmogenic nuclides, chemical weathering rates, climate. INTRODUCTION AND METHODOLOGY and soil erosion can be assumed to be negligible, long-term chemical Physical erosion and chemical weathering are interrelated pro- weathering rates can be measured using soil mass-balance techniques cesses (Stallard and Edmond, 1983) that together regulate soil depth (April et al., 1986). According to the mass-balance approach, as the and development, deliver sediment and solutes to aquatic habitats, and relatively soluble minerals in soils dissolve away, the more immobile shape mountainous landscapes. Measuring how rates of physical ero- elements should become increasingly enriched relative to their concen- sion and chemical weathering interrelate is therefore important for trations in unweathered parent material. Measurements of immobile quantitative pedology, land-use management, and landscape evolution element enrichment therefore reveal the degree of soil weathering, and modeling. Silicate weathering modulates ocean alkalinity and is thus a can be used to quantify the total dissolution loss from a soil. The long-term sink for atmospheric CO2 (e.g., Berner et al., 1983), which average weathering rate can then be estimated by dividing the disso- regulates climate via the greenhouse effect. Quantifying how climate, lution loss by the soil age. However, because non-eroding soils of weathering, and erosion are related is therefore essential for under- known age are rare, this mass-balance approach cannot be applied in standing how geomorphology and tectonics affect Earth's long-term many environments. climatic evolution (Raymo et al., 1988; Molnar and England, 1990). To the extent that chemical weathering rates increase with temperature, New Mass-Balance Approach for Measuring Weathering Rates weathering feedbacks should, over millions of years, buffer Earth's Here we show how the soil mass-balance approach can be ex- climate against large temperature shifts (e.g., Berner et al., 1983). To tended to measure long-term weathering rates in eroding landscapes. the extent that chemical weathering rates are strongly coupled with If soil formation from rock is counterbalanced by soil loss from phys- physical erosion rates (Stallard and Edmond, 1983), and thus with ical erosion and chemical weathering, then soil depth will be constant mountain uplift rates, periods of increased mountain uplift would be (e.g., Heimsath et al., 1997) and marked by global cooling, due to increased atmospheric CO2 con- sumption by weathering (Raymo et al., 1988). Psoil 5 D 5 E 1 W, (1) Previous Measurements of Chemical Weathering Rates where Psoil is the soil production rate, D is the total denudation rate, Relationships among chemical weathering, physical erosion, and E is the physical erosion rate, and W is the chemical weathering rate. climate have remained poorly quanti®ed because long-term chemical For insoluble elements like zirconium, removal by weathering should weathering rates have been dif®cult to measure. Chemical weathering be zero, so inputs from soil production will be balanced solely by rates have typically been measured from year- to decade-long records outputs from physical erosion: of solute ¯uxes, but these short-term measurements do not necessarily apply on the long time scales over which soils, landscapes, and cli- [Zr]rock3 D 5 [Zr] soil 3 E, (2) mates evolve. In the rare instances in which soil age can be determined where [Zr]rock and [Zr]soil are representative zirconium concentrations *E-mail: [email protected]. of the rock and soil. By substitution, we can rewrite equation 1 as: q 2001 Geological Society of America. For permission to copy, contact Copyright Clearance Center at www.copyright.com or (978) 750-8400. Geology; June 2001; v. 29; no. 6; p. 511±514; 2 ®gures; 1 table; Data Repository item 2001054. 511 TABLE 1. CHEMICAL DEPLETION FRACTIONS AND WEATHERING RATES* Basin Denud- [Zr]saprolite [Zr]outcrop [Zr]rock [Zr]soil Chemical Weathering ation depletion rate** rate² fraction (t´km22´yr21) (ppm)§ (ppm)§ (ppm)§ (ppm)§ (%) WSi W (t´km22´yr21) Fort Sage (granodiorite; 25 6 3 cm/yr; 12.2 6 0.6 8C; average depletion 5 15% 6 3%) A1 77 6 7 N.D. 112 6 3 (2) 112 6 3 118 6 5 (5) 6 6 506 146 4 A2(s) 56 6 15 120 6 4 (5) 112 6 3 (2) 118 6 3 139 6 5 (19) 15 6 426 196 3 A3(s) 152 6 27 N.D. 113 6 4 (4) 113 6 4 139 6 7 (16) 18 6 586 3286 9 A4(s) 680 6 211 118 6 4 (4) 122 6 3 (2) 119 6 3 142 6 3 (22) 16 6 3366 13 110 6 38 Fall River (tonalite; 145 6 5 cm/yr; 11.9 6 0.6 8C; 19% 6 2%) FR-2 393 6 82 94 6 9 (12) 85 6 6 (3) 92 6 7 109 6 6 (13) 15 6 8196 12 60 6 34 FR-5 293 6 36 121 6 5 (7) 114 6 3 (4) 119 6 3 150 6 5 (25) 21 6 3216 4616 13 FR-6 89 6 25 N.D. 83 6 5 (3) 83 6 5 102 6 3 (9) 19 6 566 2176 7 FR-8 38 6 5 N.D. 87 6 1 (3) 87 6 1 106 6 3 (9) 18 6 326 176 1 Adams Peak (granodiorite; 58 6 7 cm/yr; 4.2 6 0.5 8C; average depletion 5 14% 6 2%) AP-3 124 6 12 111 6 4 (5) 112 6 1 (3) 111 6 2 135 6 3 (20) 17 6 266 1216 4 AP-4 83 6 8 N.D. 108 6 1 (3) 108 6 1 115 6 4 (9) 6 6 416 156 3 AP-5 148 6 13 N.D. 112 6 3 (4) 112 6 3 129 6 7 (9) 12 6 656 3186 8 AP-11 90 6 12 N.D. 109 6 6 (2) 109 6 6 129 6 5 (4) 15 6 646 2146 5 AP-13 117 6 12 N.D. 112 6 5 (2) 112 6 5 131 6 3 (4) 14 6 456 2176 5 Antelope Lake (granodiorite; 83 6 6 cm/yr; 7.8 6 0.4 8C; average depletion 5 18% 6 5%) AL-4 66 6 8 N.D. 166 6 15 (4) 166 6 15 227 6 8 (9) 27 6 756 2186 5 AL-5 78 6 12 N.D. 165 6 48 (2) 165 6 48 211 6 10 (7) 22 6 23 5 6 5176 18 AL-9 98 6 13 N.D. 211 6 25 (3) 211 6 25 271 6 18 (8) 22 6 11 6 6 3226 11 AL-10 81 6 8 182 6 11 (8) 192 6 7 (2) 183 6 9 212 6 6 (26) 14 6 536 1116 4 Sunday Peak (granite; 105 6 5 cm/yr; 9.4 6 0.4 8C; average depletion 5 10% 6 4%) SP-1 108 6 12 228 6 16 (8) 214 6 7 (3) 225 6 12 252 6 7 (23) 11 6 646 2126 6 SP-3 82 6 10 N.D. 238 6 12 (3) 238 6 12 245 6 16 (9) 3 6 816 236 7 SP-8 82 6 8 N.D. 244 6 13 (4) 244 6 13 278 6 7 (8) 12 6 536 2106 4 Nichols Peak (granodiorite; 22 6 3 cm/yr; 15.4 6 0.5 8C; average depletion 5 25% 6 6%) NP-1 113 6 1 119 6 8 (5) 140 6 4 (2) 125 6 7 160 6 10 (19) 22 6 686 2246 8 NP-18 81 6 8 197 6 3 (4) 229 (1) 203 6 19 285 6 15 (14) 29 6 866 2236 7 *Site descriptions (underlined) include lithology, average precipitation, mean annual temperature, and average chemical depletion fraction (weighted by inverse variance). ²Denudation rates inferred from cosmogenic nuclide concentrations in quartz stream sediment. For methodology see supplemental information (text footnote 1). §Zr concentrations are measured by X-ray ¯uorescence and reported as averages 6 standard errors; number of samples in parentheses. [Zr]rock is averaged from the combined pool of saprolite and outcrop samples. Our procedures for sampling representative soil and rock are reported in Appendix 1 (see text footnote 1). N.D. 5 not determined. **Weathering rates (W) inferred from equation 3. See Appendix 1 (text footnote 1) for details on how we calculate WSi, the silicon chemical weathering rate. W 5 D 3 (1 2 [Zr]rock /[Zr] soil ). (3) be reasonable (see footnote 1 for further discussion).
Details
-
File Typepdf
-
Upload Time-
-
Content LanguagesEnglish
-
Upload UserAnonymous/Not logged-in
-
File Pages4 Page
-
File Size-