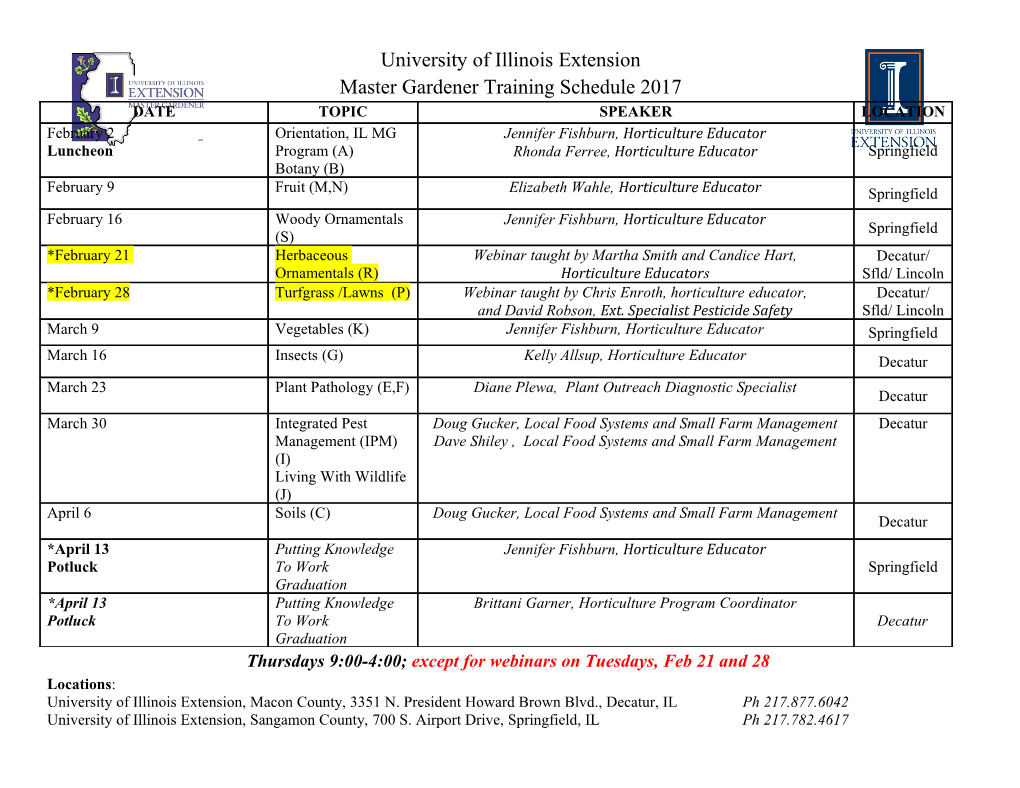
FEMS Microbiology Ecology, 97, 2021, fiaa249 doi: 10.1093/femsec/fiaa249 Advance Access Publication Date: 4 December 2020 Minireview MINIREVIEW Synthetic biology approaches to copper remediation: Downloaded from https://academic.oup.com/femsec/article/97/2/fiaa249/6021318 by guest on 30 September 2021 bioleaching, accumulation and recycling Andrea Giachino1,†, Francesca Focarelli1, Jon Marles-Wright2 and Kevin J. Waldron1,*,‡ 1Faculty of Medical Sciences, Biosciences Institute, Newcastle University, Newcastle upon Tyne, NE2 4HH, United Kingdom and 2School of Natural and Environmental Sciences, Newcastle University, Newcastle upon Tyne, NE1 7RU, United Kingdom ∗Corresponding author: Faculty of Medical Sciences, Biosciences Institute, Newcastle University, Newcastle upon Tyne, NE2 4HH, United Kingdom. Tel: (+44) 191 208 7036; E-mail: [email protected] One sentence summary: A review of current technologies in bacterial bioremediation, biorecycling and bioleaching, of copper homeostasis strategies used by bacteria, and how these could be exploited through synthetic biology for bioremediation. Editor: Marcus Horn †Andrea Giachino, http://orcid.org/0000-0002-7725-1065 ‡Kevin J. Waldron, http://orcid.org/0000-0002-5577-7357 ABSTRACT One of the current aims of synthetic biology is the development of novel microorganisms that can mine economically important elements from the environment or remediate toxic waste compounds. Copper, in particular, is a high-priority target for bioremediation owing to its extensive use in the food, metal and electronic industries and its resulting common presence as an environmental pollutant. Even though microbe-aided copper biomining is a mature technology, its application to waste treatment and remediation of contaminated sites still requires further research and development. Crucially, any engineered copper-remediating chassis must survive in copper-rich environments and adapt to copper toxicity; they also require bespoke adaptations to specifically extract copper and safely accumulate itasa human-recoverable deposit to enable biorecycling. Here, we review current strategies in copper bioremediation, biomining and biorecycling, as well as strategies that extant bacteria use to enhance copper tolerance, accumulation and mineralization in the native environment. By describing the existing toolbox of copper homeostasis proteins from naturally occurring bacteria, we show how these modular systems can be exploited through synthetic biology to enhance the properties of engineered microbes for biotechnological copper recovery applications. Keywords: copper; bioremediation; synthetic biology; bacteria; copper homeostasis INTRODUCTION (AMR; Grass, Rensing and Solioz 2011; Michels et al. 2015;Vin- cent, Hartemann and Engels-Deutsch 2016; Warnes and Keevil Copper (Cu) is a key metal resource for human activities. It is 2016). Copper nanoparticles (CuNP), in particular, are important used in applications as diverse as electronics, agriculture, farm- antimicrobials (Ruparelia et al. 2008;Liet al. 2013a; Lalitha et al. ing, brewing and winemaking and the wood industry (Cornu 2020) and chemical catalysts (Ressler et al. 2005; Vukojevic´ et al. et al. 2017). Moreover, the antibacterial properties of copper are 2005; Kimber et al. 2018; Tountas et al. 2019). used in healthcare as an alternative to antibiotic treatments, Each year, approximately 20 million metric tonnes of copper with the aim of tackling increasing antimicrobial resistance are mined worldwide (U.S. Geological Survey 2020), and most of Received: 4 August 2020; Accepted: 2 December 2020 C The Author(s) 2020. Published by Oxford University Press on behalf of FEMS. All rights reserved. For permissions, please e-mail: [email protected] 1 2 FEMS Microbiology Ecology, 2021, Vol. 97, No. 2 the copper entering the economy is lost at the end of its primary 2017; Rawlings 2002;Carranzaet al. 2009; Potysz et al. 2015; lifecycle. In the USA, only 35% of the 2019 copper supply was Zepeda et al. 2017). contributed by recovered copper scraps, the majority of which Recently, studies focusing on biorecycling of copper (and came from the pre-consumer stage (U.S. Geological Survey 2020). other metals) have yielded promising results (reviewed in Poll- Copper is also released into the environment by mining activi- mann et al. (2018)). Copper biorecovery from electrical and elec- ties, farming and agriculture (Poulsen 1998; Nicholson et al. 2003; tronic waste seems particularly favorable, as these types of Wilson and Pyatt 2007;Wightwicket al. 2008; Gillan et al. 2017; waste contain amounts of copper comparable to ores from tra- Lamichhane et al. 2018), and the issue of copper contamination is ditional mines, or even higher (Thakur and Kumar 2020). A particularly pressing in low-income countries (Wong et al. 2007; Strengths, Weaknesses, Opportunities and Threats (SWOT) anal- Liu et al. 2015;Singhet al. 2020). Remediation of copper pollu- ysis, published this year by Gomes, Funari and Ferrari (2020), tion will contribute to a number of the UN Sustainable Devel- identified ashes from municipal waste incineration as a suitable opment Goals, in particular Goal 6: Clean Water and Sanita- target for commercial copper recovery via bioleaching. Other tion; and Goal 9: Industry Innovation and Infrastructure (United reviews extensively addressed recent advances in the biomin- Downloaded from https://academic.oup.com/femsec/article/97/2/fiaa249/6021318 by guest on 30 September 2021 Nations General Assembly 2015). In addition, waste copper con- ing of complex metal waste, including copper (Pollmann et al. stitutes a growing above-ground resource, which could poten- 2018; Sethurajan, van Hullebusch and Nancharaiah 2018;Auer- tially complement mineral resources as high quality geological bach et al. 2019; Srichandan et al. 2019). However, the current deposits become depleted (Dunbar 2017). technology readiness level (TRL) for bioremediation and biorecy- Agricultural lands, soil and marine environment are espe- cling of copper-containing waste is still low (Gomes, Funari and cially threatened by copper pollution since this metal is a pow- Ferrari 2020), partially owing to current low mining costs and erful pollutant even at moderate concentrations (Rehman et al. cheap landfill disposal (Gomes, Funari and Ferrari 2020). This is 2019;Singhet al. 2020). However, what constitutes a ‘safe’ level expected to change rapidly in the future (Dunbar 2017), as biore- of copper concentration is currently unclear. The World Health mediation of contaminated sites becomes more pressing. Organization guidelines define 2 mg/L as the safe upper limit for One crucial challenge in copper recovery is the toxic nature of total dissolved copper levels in water (WHO 1993, 2004). How- this ion. Most biomining and bioremediating strains are selected ever, the natural copper content in soil is hugely variable, rang- from environmental samples that survive in copper-rich envi- ing from tens to thousands of mg Cu/kg depending on local geol- ronments (Orell et al. 2010); and in the case of biomining, a well- ogy and anthropogenic activities (Cornu et al. 2017; Gillan et al. established suite of methods is available to recover, test and 2017; Rehman et al. 2019). Freshwater and marine ecosystems select bioleaching organisms (Schippers 2007;Orellet al. 2010; are similarly variable, and may experience seasonal fluctuations Gumulya et al. 2018; Pourhossein and Mousavi 2018). On the in the range of μg–mg/L (Miller et al. 1966; Boyle, Huested and other hand, the use of synthetic biology in copper recovery is a Jones 1981; Kremling and Hydes 1988; Nagorski, McKinnon and relatively recent concept (Capeness and Horsfall 2020). Nonethe- Moore 2003; Belabed et al. 2017). less, some synthetic biology applications have been explored As environmental copper concentrations are highly variable, for biomining (Dunbar 2017; Gumulya et al. 2018) and post- so is the copper tolerance of local microorganisms. The majority production remediation (Capeness and Horsfall 2020). Develop- of bacteria require only trace quantities of copper to metalate ments in this field face technical challenges, and also the eth- their copper-dependent enzymes (Festa and Thiele 2011), and ical and legal issues created by the potential for environmen- can efficiently scavenge this essential nutrient. Those organisms tal release of genetically modified organisms (Das, Dash and that feature unusually high copper demands, such as methan- Chakraborty 2016). otrophic bacteria, have evolved to increase the affinity of their In this review, we summarize existing applications of bio- copper acquisition systems rather than migrate to copper-rich logical systems for bioremediation, bioleaching and biomining. environments (El Ghazouani et al. 2012). Indeed, most bacteria, We then describe the advantages of genetically modified bac- including common pathogens, are sensitive to copper at μMlev- teria for these applications and review the available toolkit for els (Rensing and Grass 2003; Tottey et al. 2012;Tarrantet al. 2019). copper-related synthetic biology in bacteria. This includes bac- These microbes, however, can evolve to tolerate copper concen- terial strains, copper-handling pathways and copper biosensors trations that are orders of magnitude higher, typically through that can be exploited to detect, capture and re-utilize copper duplication of copper resistance genes or horizontal transfer of from different environments. We show how these tools
Details
-
File Typepdf
-
Upload Time-
-
Content LanguagesEnglish
-
Upload UserAnonymous/Not logged-in
-
File Pages14 Page
-
File Size-