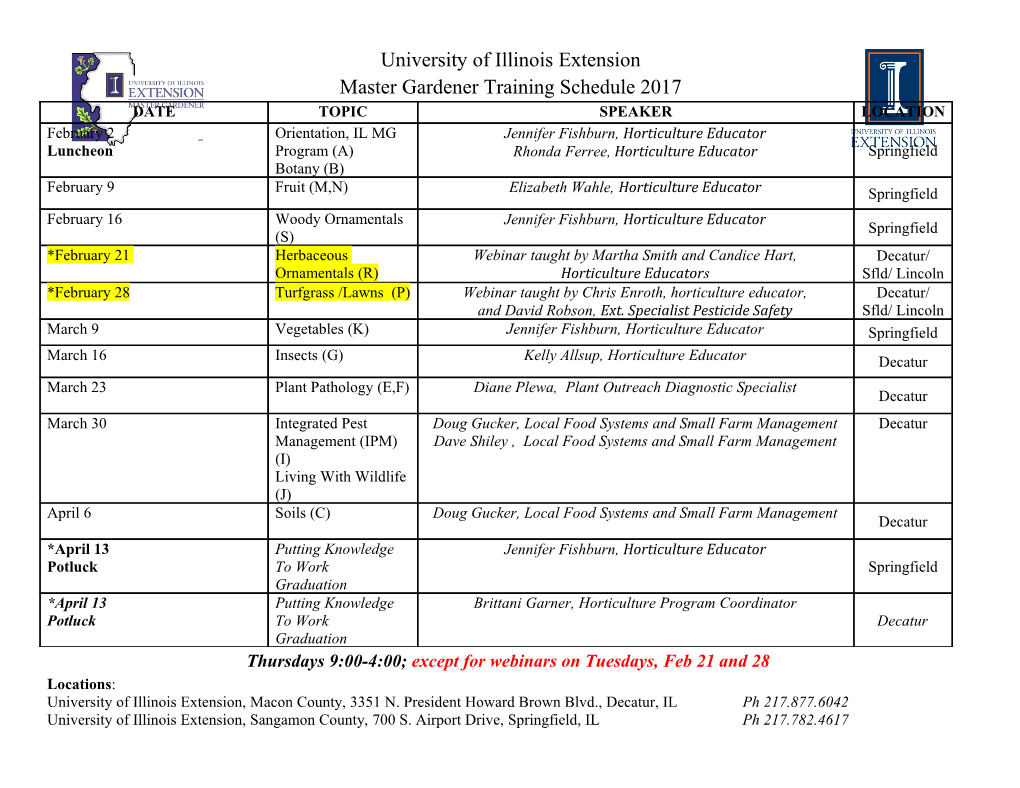
University of South Florida Scholar Commons Graduate Theses and Dissertations Graduate School June 2020 Genome Maintenance Roles of Polycomb Transcriptional Repressors BMI1 and RNF2 Anthony Richard Sanchez IV University of South Florida Follow this and additional works at: https://scholarcommons.usf.edu/etd Part of the Molecular Biology Commons Scholar Commons Citation Sanchez, Anthony Richard IV, "Genome Maintenance Roles of Polycomb Transcriptional Repressors BMI1 and RNF2" (2020). Graduate Theses and Dissertations. https://scholarcommons.usf.edu/etd/8485 This Dissertation is brought to you for free and open access by the Graduate School at Scholar Commons. It has been accepted for inclusion in Graduate Theses and Dissertations by an authorized administrator of Scholar Commons. For more information, please contact [email protected]. Genome Maintenance Roles of Polycomb Transcriptional Repressors BMI1 and RNF2 by Anthony Richard Sanchez IV A dissertation submitted in partial fulfillment of the requirements for the degree of Doctor of Philosophy Department of Cell, Micro, and Molecular Biology College of Arts and Sciences University of South Florida Major Professor: Younghoon Kee, Ph.D Kristina Schmidt, Ph.D Meera Nanjundan, Ph.D Gary Daughdrill, Ph.D. Date of Approval: June 17, 2020 Keywords: DNA Damage, Genomic stability, Replication, Transcription Copyright© 2020, Anthony Richard Sanchez IV Dedication I would like to dedicate this dissertation to my caring and supportive (soon to be) wife Diamond Sprague, she has always been here for me during my best and worst times throughout graduate school. I would also like to dedicate this to my parents, their love and devotion shaped me into who I am today and inspires me to keep growing. Acknowledgments Firstly, I would like to thank Dr.Younghoon Kee for all of his guidance and mentorship throughout my time in graduate school. His exemplifies how to be a successful researcher and citizen of the scientific community. The tools and experience I have gained under his leadership will benefit me for the rest of my career. I would also like to thank all of the Kee lab members for their help along the way, particularly Angelo de Vivo for always challenging my ideas and making significant scientific contributions to this work. Also, our former post doc Dr. Jeonghyeon Kim for developing the ChIP assay in our lab and setting an excellent example of how to be a successful post-doc. I thank all of my committee members, Drs. Kristina Schmidt, Meera Nanjundan, and Gary Daughdrill for all of their helpful input throughout the development of this work. They provided me with the essential experience of justifying my scientific choices and learning how to critically examine my own work. Throughout my time in graduate school I also received a tremendous amount of help from Robert Hill, MS. From teaching me how to use a confocal microscope to helping fix equipment or just being there to trouble shoot with, Robert was always available when I needed him. Throughout the course of this work I had the privilege to collaborate with many great researchers, I would like to thank all of my co-authors for their contributions and help along the way. I would like to thank Dr.Stanley Stevens Jr. and Dr.Dale Chaput at the University of South Florida’s Center for Drug Discovery and Innovation proteomics facility for Mass Spec analysis. Several scientists assisted in the 2016 paper by contributing reagents, I thank Dr. Roger Greenberg for the PTuner263 cell line, Dr. Bert Vogelstein for the HCT116 p21−/− cell line and Dr. Charles Watts for sharing the pCMV–Tag2B–UBR5 plasmid through Addgene. I would also like to thank all of the researchers who contributed reagents for the 2020 paper, Dr. Meera Nanjundan for providing T80 cells, the pyCAG_RNaseH1_WT and D210N plasmids were gifts from Dr. Xiang-Dong Fu through Addgene. I also thank Dr. Charles Szekeres for technical support for Flow cytometry and Dr. Catherine Freudenreich for helpful insight and suggestions regarding common fragile sites. Table of Contents List of Tables iii List of Figures iv Abstract vi Chapter one: Introduction 1 Preface 1 Structure and Functions of PRC1 2 Histone H2A 4 Non-canonical functions of BMI1 and RNF2 6 Functions of UBR5 9 Functions of the FACT Complex 12 Relevance of Common Fragile Sites 13 The Role of Transcription-Replication Conflicts in Genomic Stability 14 Rational and Hypothesis 18 Chapter two: The BMI1-UBR5 Axis Regulates Transcriptional Silencing at Sites of DNA Damage 21 Methods 21 Cell lines, plasmids and chemicals 21 RNAi 22 Western blot analysis 22 Immunoprecipitation and mass spectrometry analysis 23 Immunofluorescence and image quantification 24 Proximity ligation assay 25 pTuner transcriptional reporter assay 26 In vitro ubiquitination assay 26 Cell fractionation assay 26 Clonogenic survival assay 27 Histone H2A Exchange assay (SNAP) 27 Results 28 UBR5 chromatin localization is dependent on BMI1, RNF1, and RNF2 28 UBR5 is a regulator of transcription at damaged chromatin 32 UBR5 associates with BMI1 and the FACT complex 35 BMI1 and UBR5 regulate FACT-mediated transcriptional elongation at UV-induced lesions 37 Discussion 42 Summary of Findings 42 i Novel functions of UBR5 43 Novel functions of the FACT complex 44 Novel functions of BMI1 45 Chapter Three: Replication-Transcription Conflicts as a Source of Common Fragile Site Instability Caused by BMI1-RNF2 Deficiency 47 Methods 47 Cell lines, plasmids, and chemicals 47 RNAi 48 Western Blots and antibodies 48 Immunofluorescence and image quantification 49 Proximity ligation assay 50 Chromatin immunoprecipitations 50 qPCR 51 Clonogenic survival assay 52 Cell cycle analysis 52 Results 53 Depletion of BMI1 or RNF2 causes replication fork stresses 53 Depletion of BMI1/RNF2 leads to CFS instability 56 Depletion of BMI1 or RNF2 causes transcription stress at CFSs and transcription-replication collisions 58 Fanconi Anemia proteins respond to R-loop-associated transcriptional stress in RNF2-deficient cells 60 Discussion 65 Summary of findings 65 Coordination of transcription and replication 66 A novel relationship between Fanconi Anemia and Polycomb proteins 68 Chapter Four: Conclusions and Future Directions 70 Conclusions 70 Future Outlook 71 The relationship between UBR5 and BMI1 71 The role of H2A ubiquitination by RNF2 73 Clinical Implications and broader impact 76 Works Cited 79 Appendix I: Supplemental Information 85 ii List of Tables Table 1: siRNA used in Chapter 2 22 Table 2: Antibodies used in Chapter 2 22 Table 3: siRNA used in Chapter 3 48 Table 4: Antibodies used in Chapter 3 48 Table 5: Primer Sequences used in ChIP Experiments 52 Supplemental Table 1: gRNA sequences used in chapter 3 93 iii List of Figures Figure 1: Model of PRC1 involvement in development 3 Figure 2: Model of potential outcomes for lack of transcriptional repression 16 Figure 3: Model of PRC1 involvement during the DDR 19 Figure 4: UBR5 is recruited to DNA damage 29 Figure 5: UBR5 Recruitment to damage is dependent on BMI1 and RNF2 31 Figure 6: UBR5 and BMI1 regulate transcription at damage sites 33 Figure 7: UBR5 associates with BMI1 and SPT16 36 Figure 8: BMI1 and UBR5 regulate FACT Chromatin localization 40 Figure 9: UBR5 and OTUD5 influence Histone H2A deposition 41 Figure 10: UBR5 and BMI1 are needed for cellular homeostasis 42 Figure 11: Model of transcriptional repression by UBR5 and BMI1 43 Figure 12: Global indicators of replication stress is increased with RNF2 or BMI1 Depletion 55 Figure 13: Replication associated damage is increased at CFS with RNF2 depletion 57 Figure 14: Active transcription at CFS is increased with RNF2 depletion 59 Figure 15: TRC is increased in BMI1 and RNF2 Depleted cells 60 Figure 16: R-loops are increased in the absence of BMI1 and RNF2 62 Figure 17: R-loops generated in the absence of BMI1 and RNF2 are responded to by the ID2 complex 64 Figure 18: Model of CFS protection by BMI1 and RNF2 66 Figure 19: Complete model of signaling network presented 71 Figure 20: The RNF2 I53A mutation does not disrupt the regulation of transcription 75 Supplemental Figure 1: Conceptual Model of the Proximity Ligation Assay (PLA) 85 Supplemental Figure 2: Schematic of transcriptional reporter assay in pTuner 263 cells 86 Supplemental Figure 3: Knockdown efficiency for BMI1 in HELA cells. 87 Supplemental Figure 4: Knockdown efficiency for BMI1, RNF1 and RNF2 in HELA cells. 87 Supplemental Figure 5: Control knockdowns for RNAPII/γH2AX experiments 88 iv Supplemental Figure 6: Phenotypes related to UBR5 catalytic activity 89 Supplemental Figure 7: Benzonase treated SPT16 IP/WB 90 Supplemental Figure 8: Single antibody controls for PLA shown in Figure 7G 91 Supplemental Figure 9: SPT16 and SSRP1 knockdowns in UBR5 KO cells 92 Supplemental Figure 10: Western blot for RNF2 expression in T80 KO cells 93 Supplemental Figure 11: Knockdown efficiencies for RNF2, BMI1 and TOP2A in U2OS 94 Supplemental Figure 12: BMI1 and RNF2 are expressed in the nucleus of replicating cells 95 Supplemental Figure 13: Western blot for V5 (RNH1 D210N) IP efficiency 96 Supplemental Figure 14: Sequencing for RNF2 I53A U2OS cell line 97 v Abstract The coordination of transcription, replication, and DNA damage response (DDR) is vital for maintaining normal cellular homeostasis. All of these processes take place on the chromatin and thus, the temporal and spatial separation of the factors responsible are necessary for each to be correctly completed. Here we detail several novel processes contributing to this network. BMI1 is a component of the Polycomb Repressive Complex 1 (PRC1) which plays a key role in maintaining epigenetic silencing programs during development. Recently, BMI1 and other members of PRC1 like RNF2 have been implicated gene silencing during the DDR; however, the mechanism through which BMI1 and RNF2 impose this transcriptional repression is still under investigation.
Details
-
File Typepdf
-
Upload Time-
-
Content LanguagesEnglish
-
Upload UserAnonymous/Not logged-in
-
File Pages109 Page
-
File Size-