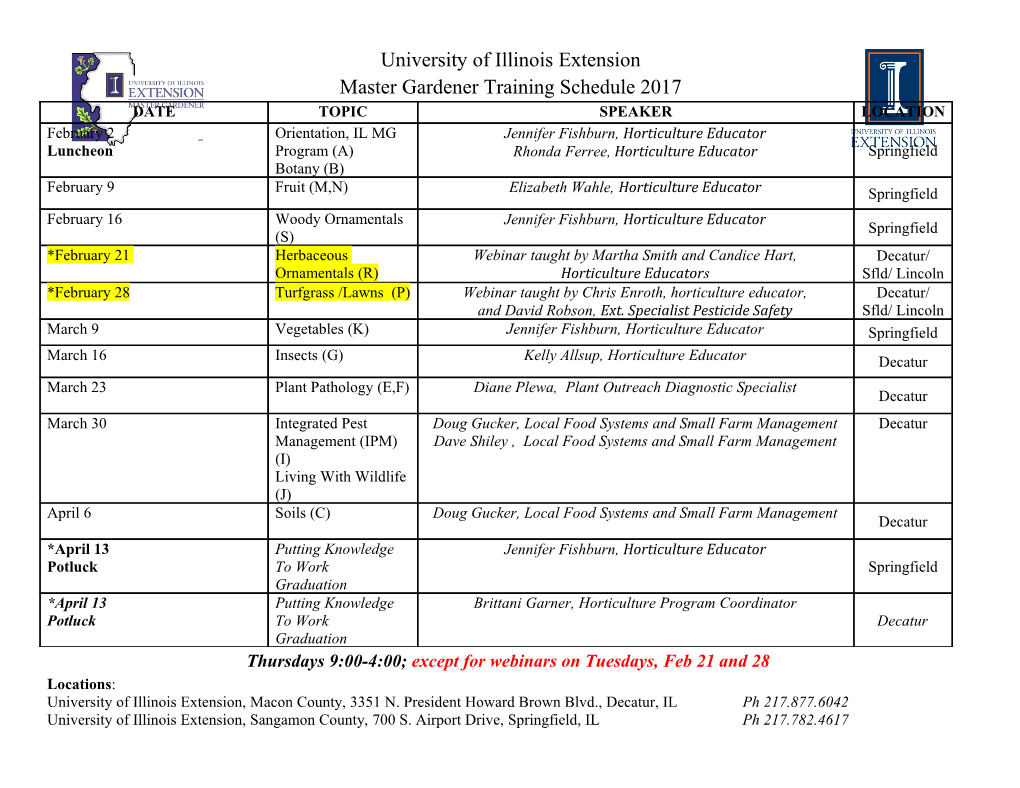
Table of contents Genomic conflicts in Podospora anserina MARIJN VAN DER GAAG 1 Promotor: Prof. Dr. Rolf F. Hoekstra Hoogleraar in de Genetica Wageningen Universiteit Copromotor: Dr. Ir. Alfons J. M. Debets Universitair Hoofddocent Laboratorium voor Erfelijkheidsleer Wageningen Universiteit Promotiecommissie: Dr. Sven J. Saupe, Laboratoire Génétique Moleculaire des Champignons, Bordeaux, France Prof. Dr. (Kuke) R. Bijlsma, Rijksuniversiteit Groningen Prof. Dr. Piet Stam, Wageningen Universiteit Prof. Dr. Pedro W.Crous, Centraal Bureau voor Schimmelcultures, Utrecht en Wageningen Universiteit Dr. Ir. A. J. Termorshuizen, Wageningen Universiteit 2 Table of contents Genomic conflicts in Podospora anserina Genomische conflicten in Podospora anserina Marijn van der Gaag Proefschrift ter verkrijging van de graad van doctor op gezag van de rector magnificus van de Wageningen Universiteit, Prof. Dr. M. J. Kropff in het openbaar te verdedigen op woensdag 19 oktober 2005 des namiddags te vier uur in de Aula. 3 van der Gaag, Marijn Genomic conflics in Podospora anserina / Marijn van der Gaag Thesis Wageningen University, with references – and summary in Dutch. ISBN 90-8504-255-0 Subject headings: fungal genetics / linear plasmid / meiotic drive / Podospora anserina / spore killing. 4 Table of contents Voor Alarik en Anne 5 6 Table of contents Table of Contents Chapter 1 General Introduction Page 9 Chapter 2 The dynamics of pAL2-1 homologous linear plasmids in Page 23 Podospora anserina . Chapter 3 Spore killing: Meiotic drive factors in a natural population of Page 35 the fungus Podospora anserina . Chapter 4 Spore killing in the fungus Podospora anserina : a connection Page 55 between meiotic drive and vegetative incompatibility? Chapter 5 Possible mechanisms of spore killing in Podospora anserina. Page 63 Chapter 6 Sexual compatibility and outcrossing in Podospora anserina. Page 83 Chapter 7 General Discussion. Page 103 Literature cited. Page 121 Summary Page 141 Samenvatting Page 145 Nawoord Page 149 Curriculum vitae Page 151 7 8 General Introduction Chapter 1 General Introduction “Mendelism is a magnificent invention for fairly testing genes in many combinations, like an elegant factorial experimental design. Yet it is vulnerable at many points and is in constant danger of subversion by cheaters that seem particularly adept at finding such points.” - J. F. Crow (1988) In the next paragraphs the definition of genomic conflicts is introduced, followed by two examples of such conflicts that (also) exist in the ascomycete fungus Podospora anserina. These are genomic conflicts based on transfer of (parasitic) genetic elements and genomic conflicts based on deviations from Mendelian inheritance, also known as meiotic drive. Then characteristics of the fungus itself followed by several aspects of Podospora that influence these genomic conflicts are discussed. Finally, the outline of this thesis is described. GENOMIC CONFLICTS … The general view of the genome of an organism is an integrated and cooperative network of genes that is both viable and reproductive. Mutations in the genome that decrease the fitness of the organism are selected out, whereas mutations with beneficial effects on the fitness of the organism and its genome become established. However, a large proportion of the genome of eukaryotes is composed of selfish genetic elements (Hurst and Werren 2001). Selfish or ‘parasitic’ elements promote their own transmission relative to the other elements of the genome, but have neutral or even detrimental effects on the fitness of the organism. Hurst and Werren (2001) divide these common but diverse elements into four categories, based upon the mechanism by which they spread. These are: - Autonomously replicating (mobile) elements, such as transposons and many fungal mitochondrial plasmids (Griffiths 1995). - (Post) segregation distorters like meiotic drive elements, B chromosomes and killer plasmids. - Sex-ratio distorters like cytoplasmic microorganisms that feminize hosts or kill males. - Gene converting elements, such as homing endonucleases. A genetic conflict, where the spread of one gene creates the context for the spread of another gene having the opposite effect and expressed in the same individual may result in a 9 Chapter 1 genomic conflict. A genomic conflict arises when the effects of these selfish elements are opposite to the interests of the other parts of the genome. Basically, genetic conflicts are intra- individual, but become genomic when more (closely linked) genes become involved (Hurst et al. 1996). Genomic conflicts are evolutionary interesting, because they can give insight in why or how certain genetic features have arisen. For instance meiotic drive elements can offer perspectives why ‘fair’ Mendelian inheritance is stable and to observe the evolution of mechanisms that counteract drive (Lyttle 1991, 1993). Recombination (random assortment of chromosomes and crossing over) might have its origin or is maintained to act against distorter genes by minimizing the size of linkage groups and hence to prevent the formation of potential drive allegiances by leaving the majority of genes freely recombining (Eshel 1985, Haig and Grafen 1991). Selfish elements are speculated to have influence on speciation by horizontal gene transfer (Rosewich and Kistler 2000) or by contributing to reproductive isolation, e.g. due to chromosomal rearrangements caused by segregation distorters or transposons or cytoplasmic incompatibility systems (Hurst and Werren 2001, Hurst et al. 1996). Furthermore, the genetic conflict between Mendelian nuclear genes and non- Mendelian sex-ratio distorters might have a role in the great variety of sex determination systems in plants and animals. According to Hurst and Werren (2001) selfish genetic elements obey two common rules. The first rule is that the diversity of selfish genetic elements in a species is correlated with the rate of outcrossing. Inbreeding decreases the heterozygosity and lowers the opportunity for transmission between genomes. This also affects the possibilities for effective selfish behavior and accordingly the spread of the selfish element. The second rule is that their phenotype is often shown in hybrids and between- population crosses, but that they are seldom observed in within-population crosses. The outcome of a genomic conflict within a population would either lead to removal or fixation of the selfish element, or even complete annihilation of the population (Hurst et al. 1996). A between-population cross can restore the genetic diversity for the element in question, resulting in renewed expression. To observe a genomic conflict within a population would mean that the conflict is not yet resolved or a condition of stalemate, as within SD of Drosophila , is reached (Hurst et al. 1996). Fast termination of a conflict rarely produces any long-lasting effects, whereas extension of the conflict would provide more opportunities for modifiers, as well as secondary effects to appear. Suppressors are generally not cost-free and may not be restricted to counteracting the selfish element. A modifying allele can also be a neutral party and therefore not increase in frequency. Reappearance of conflicts can lead to a decrease in vulnerability to the action of selfish elements due to suppressors and modifiers of previous conflicts. For instance modifiers that increase recombination rate, would hamper spread of future drive elements (Hurst et al. 1996). Two examples of selfish elements used in this study, meiotic drive elements and (fungal) plasmids are discussed in more detail below. 10 General Introduction Meiotic Drive (selfish chromosomal elements) Meiotic drive is the mechanism by which one of the members of a pair of heterozygous alleles or chromosomes during meiotic divisions is transmitted into the progeny in excess of the expected ‘fair’ Mendelian proportion of 50%. Segregation distorters, the elements that perform meiotic drive are found in a wide range of organisms and usually involve a small number of interacting loci. Drive elements are coined ‘ultraselfish’ DNA, because they increase their frequency by actively promoting the destruction of the alternative allele (Crow 1988, Lyttle 1991). Segregation advantage arises at the population level, not at the individual level, where competition occurs among the gametes of single individuals (as in fungal drive systems, where the gametes are the offspring), and gamete loss results in less than proportional loss of individual fecundity. Logically, segregation distorter systems are more likely to be found in males of monogamous species, as the impact of gamete wastage is smallest (Lyttle 1991). Segregation distorters can be divided into two groups, autosomal or sex-linked. Well-studied examples of sex-ratio distortion are X chromosome drive (SR) in Drosophila and male drive (MD) in mosquitoes. Examples of autosomal drive are the t- haplotypes in mice, segregation distorter (SD) in Drosophila and Spore killer ( Sk, Psk ) in fungi (Lyttle 1991, Turner and Perkins 1991, Nauta et al. 1993). Meiotic drive is initially limited in impact to population dynamics of the drive locus and those loci in close linkage. Alleles at these latter loci may exhibit indirect drive through genetic hitchhiking, and increase in frequency even though they might have negative effects on the individual fitness. Meiotic drive can also lead to loss of fecundity through gamete loss, as is the case with fungal drive systems (Nauta and Hoekstra 1993), or through
Details
-
File Typepdf
-
Upload Time-
-
Content LanguagesEnglish
-
Upload UserAnonymous/Not logged-in
-
File Pages154 Page
-
File Size-