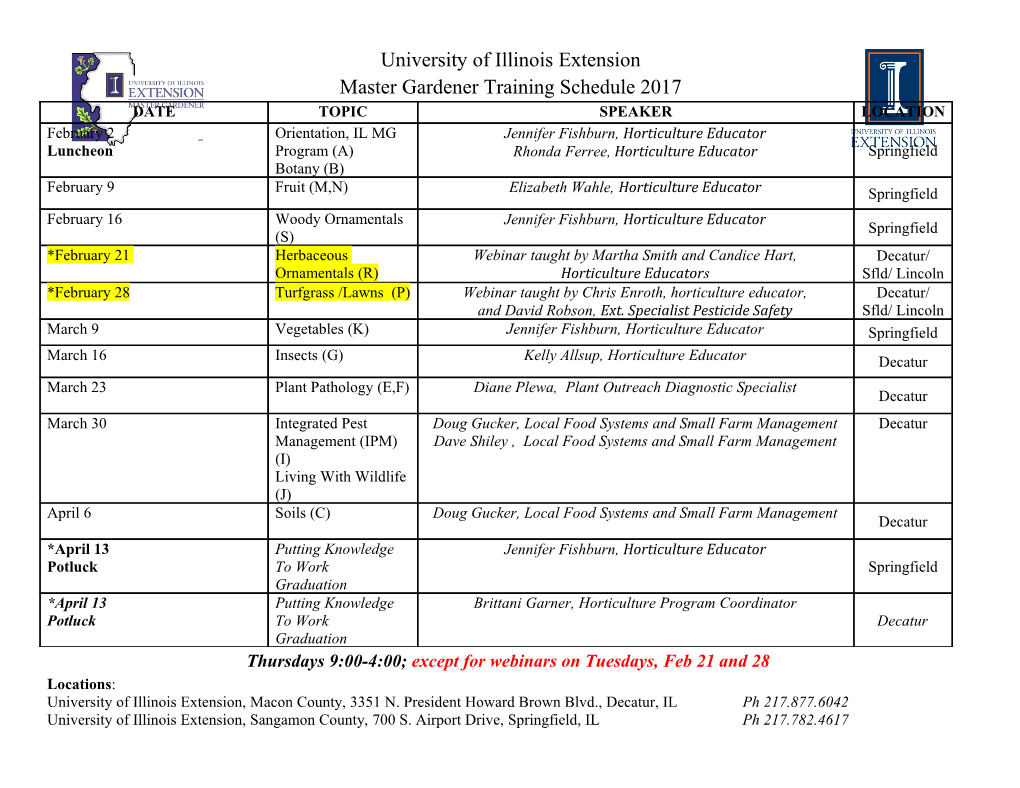
Adiabatic quantum-flux-parametron with delay-line clocking: logic gate demonstration and phase skipping operation Taiki Yamae,1,2 Naoki Takeuchi,3,4,* and Nobuyuki Yoshikawa1,4 1 Department of Electrical and Computer Engineering, Yokohama National University, 79-5 Tokiwadai, Hodogaya, Yokohama 240-8501, Japan 2 Research Fellow of Japan Society for the Promotion of Science, 5-3-1 Kojimachi, Chiyoda, Tokyo 102-0083, Japan 3 Research Center for Emerging Computing Technologies, National Institute of Advanced Industrial Science and Technology (AIST), 1-1-1 Umezono, Tsukuba 305-8568, Japan 4 Institute of Advanced Sciences, Yokohama National University, 79-5 Tokiwadai, Hodogaya, Yokohama 240-8501, Japan * [email protected] Abstract. Adiabatic quantum-flux-parametron (AQFP) logic is an energy-efficient superconductor logic family. The latency of AQFP circuits is relatively long compared to that of other superconductor logic families and thus such circuits require low-latency clocking schemes. In a previous study, we proposed a low-latency clocking scheme called delay-line clocking, in which the latency for each logic operation is determined by the propagation delay of the excitation current, and demonstrated a simple AQFP buffer chain that adopts delay-line clocking. However, it is unclear whether more complex AQFP circuits can adopt delay-line clocking. In the present study, we demonstrate AQFP logic gates (AND and XOR gates) that use delay-line clocking as a step towards implementing large-scale AQFP circuits with delay-line clocking. 1 AND and XOR gates with a latency of approximately 20 ps per gate are shown to operate at up to 5 and 4 GHz, respectively, in experiments. We also demonstrate that delay-line clocking enables phase skipping operation, in which some of the AQFP buffers for phase synchronization are removed to reduce the junction count and energy dissipation. The results indicate that delay-line clocking can enable low-latency, low-energy, large-scale AQFP circuits. 2 1. Introduction Josephson junctions can be used as superconducting switching devices with a few-picosecond characteristic time and a sub-aJ energy barrier height. Superconducting digital circuits based on Josephson junctions [1–4] can thus achieve high operating speed and low switching energy, making them a key technology for developing superconducting information systems, such as microprocessors [5–8] and non-Neumann computing systems [9–12]. To realize large-scale, high-performance superconducting systems, fabrication processes have been continuously improved [13–15] and many types of circuit design tool have been developed [16–18]. Adiabatic quantum-flux-parametron (AQFP) logic [19] is an energy-efficient superconductor logic family based on the quantum flux parametron [20, 21]. Because adiabatic switching [22, 23] enables a logic gate to switch without rapid state transition, the switching energy of an AQFP gate can be reduced to less than the Josephson energy [24]. Recently, we designed and demonstrated the 4-bit Monolithic Adiabatic iNtegration Architecture (MANA) microprocessor that uses AQFP logic [8], thereby proving the high robustness and high energy efficiency of AQFP circuits. We found that the latency of this microprocessor is relatively long (for instance, 5400 ps for a 5-GHz clock frequency). The reason for this long latency is that the microprocessor is driven by four-phase clocking [25, 26], in which AQFP circuits are driven by a pair of excitation currents with a phase separation of 90° and a latency of a quarter clock cycle (50 ps at 5 GHz) per gate is required. To reduce the latency of AQFP microprocessors, it is necessary to adopt low-latency clocking schemes such as delay-line clocking [27], in which the latency of each logic operation is determined by the propagation delay of the excitation current. In a previous study, we demonstrated an AQFP buffer chain with a latency of approximately 10 ps per gate achieved using delay-line clocking [27]. This suggests that the latency of large-scale AQFP circuits, such as microprocessors, can be significantly reduced by adopting 3 delay-line clocking. In the present study, we conduct a simulation and experiments as a step toward implementing large-scale AQFP circuits with delay-line clocking. Specifically, we show that (i) delay-line clocking can be used to operate AQFP logic gates with low latency and that (ii) delay-line clocking can reduce the number of buffers required for phase synchronization. Because delay-line clocking adoption has been limited to buffers [27], we first demonstrate AQFP logic gates (AND and XOR gates) that use delay-line clocking to show that delay-line clocking is applicable to complex AQFP circuits. Then, we show that delay-line clocking can remove some buffers required for phase synchronization, i.e., it enables the skipping of some excitation phases. This is an important advantage of delay-line clocking because large-scale AQFP circuits typically require many additional buffers for phase synchronization [28]. Finally, we fabricate and operate a test circuit to demonstrate these properties at high clock frequencies. 2. Logic gate demonstration Figure 1(a) shows a schematic diagram of an AQFP circuit that adopts delay-line clocking for numerical simulation, where the circuit under test (CUT) is surrounded by peripheral AQFP buffers that prevent unwanted interaction with the input ports and output termination. Here, the CUT is an XOR gate. The entire circuit is powered and clocked by the excitation current Ix, which applies an ac magnetic flux with an amplitude of 0.5Φ0 to each gate. The dc offset current Id applies a dc magnetic flux of 0.5Φ0 to each gate. Ix and Id flow through the AQFP gates and delay lines, which are 50-Ω transmission lines, and are terminated by 50-Ω resistors. In this way, logic operations are performed at the rising edge of Ix with a latency of T per gate, where T is the propagation delay of each delay line. In the numerical simulation, the device parameters are based on the AIST 10 kA cm-2 Nb high-speed standard process (HSTP) [26]. The numerical 4 simulation is conducted using the Josephson circuit simulator JSIM [29]. Figure 1(b) shows transient analysis results for an XOR gate at 5 GHz for T = 10 ps, where Ista, Istb, and Istq are the signal currents that represent the logic states of buffers A, B, and Q in figure 1(a), respectively. Note that the XOR gate is composed of splitters, AND gates, and an OR gate, and thus requires three excitation phases. Hence, seven excitation phases are included between gates A (B) and Q, so that the latency between Ista (Istb) and Istq is 70 ps. This figure shows that the XOR gate operates correctly with a latency of 10 ps per gate. (a) 5 (b) Figure 1. (a) Schematic diagram of an AQFP circuit that uses delay-line clocking for numerical simulation. (b) Transient analysis results for an XOR gate operating at 5 GHz for T = 10 ps. We investigated the operating margins for an AND gate and an XOR gate that use delay-line clocking to show that AQFP logic gates can operate with wide operating margins for small T. Figure 2 shows numerical simulation results of the operating margins in terms of excitation power Px (i.e., the power of Ix) applied to AND and XOR gates as a function of T at 5 GHz. The results for a buffer are also shown for comparison. The enclosed regions represent the operation regions of the logic gates. Each logic gate was simulated using the schematic shown in figure 1(a). Figure 2 shows that both AND and XOR gates operate with wide operating margins for small T, as is the case for a buffer. Of note, the XOR gate achieves wide operating margins even though it is composed of multiple logic gates (i.e., splitters, AND gates, and an OR gate) that comprise different building blocks [30]. This suggests that delay-line clocking is applicable to complex AQFP circuits. 6 Figure 2. Simulation results of operating margins for a buffer, an AND gate, and an XOR gate that use delay-line clocking as a function of T at 5 GHz. We also calculated the energy dissipation of AND and XOR gates to show that AQFP logic gates that adopt delay-line clocking can achieve both low energy dissipation and low latency. Figure 3 shows the numerical simulation results of the energy dissipation per operation E for AND and XOR gates with delay-line clocking as a function of T for an operating frequency of 5 GHz. The results for a buffer are also shown. The energy dissipation was calculated by integrating the product of the excitation current and voltage across the excitation inductor in each gate over time; more details can be found in the literature [31]. Because the energy dissipation of AQFP circuits depends on input data combinations [32], the energy dissipation for AND and XOR gates shown in figure 3 is the average among all input data combinations. Figure 3 shows that E does not change significantly for a T value of between 10 and 50 ps for both AND and XOR gates, indicating that AQFP logic gates that adopt delay-line clocking can operate with small energy dissipation and a latency that is much less than that for conventional four-phase clocking (50 ps at 5 GHz). For a T value of less than 10 ps, E increases 7 significantly as T decreases for all logic gates. This is because for very small T, each AQFP gate switches before the previous gate is fully excited and the amplitude of the input current becomes sufficiently large [27]. Figure 3. Simulation results of energy dissipation for a buffer, an AND gate, and an XOR gate that use delay-line clocking as a function of T at 5 GHz.
Details
-
File Typepdf
-
Upload Time-
-
Content LanguagesEnglish
-
Upload UserAnonymous/Not logged-in
-
File Pages20 Page
-
File Size-