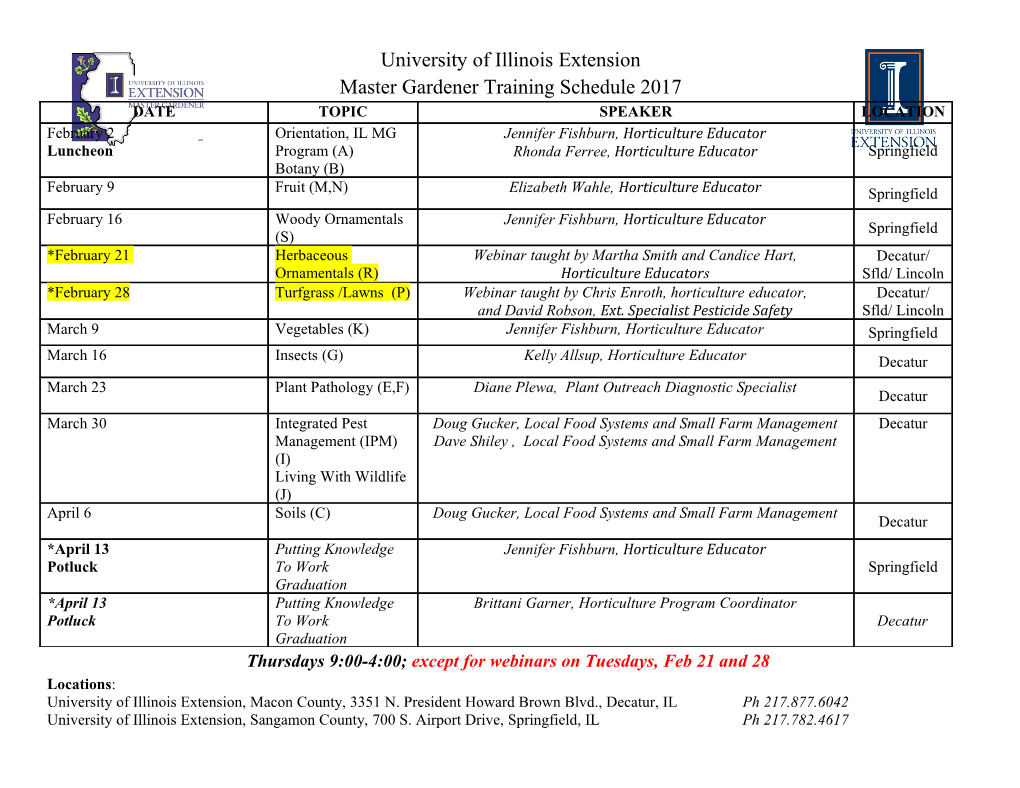
Observational Properties of Thermonuclear Supernovae Saurabh W. Jha1;2, Kate Maguire3;4, Mark Sullivan5 August 7, 2019 authors’ version of Nature Astronomy invited review article final version available at http://dx.doi.org/10.1038/s41550-019-0858-0 1Department of Physics and Astronomy, Rutgers, the State University on the explosion mechanism: objects where the energy released of New Jersey, Piscataway NJ, USA 2Center for Computational As- in the explosion is primarily the result of thermonuclear fusion. 3 trophysics, Flatiron Institute, New York, NY, USA Astrophysics Re- Given our current state of knowledge, we could equally well call search Centre, School of Mathematics and Physics, Queen’s Uni- versity Belfast, UK 4School of Physics, Trinity College Dublin, Ire- this a review of the observational properties of white dwarf su- land 5School of Physics and Astronomy, University of Southampton, pernovae, a categorisation based on the kind of object that ex- Southampton, SO17 1BJ, UK plodes. This is contrasted with core-collapse or massive star su- pernovae, respectively, in the explosion mechanism or exploding object categorisations. Unlike those objects, where clear obser- vational evidence exists for massive star progenitors and core- The explosive death of a star as a supernova is one of the collapse (from both neutrino emission and remnant pulsars), the most dramatic events in the Universe. Supernovae have an direct evidence for thermonuclear supernova explosions of white outsized impact on many areas of astrophysics: they are dwarfs is limited4,5 and not necessarily simply interpretable6,7 . major contributors to the chemical enrichment of the cos- Nevertheless, the indirect evidence is strong, though many open mos and significantly influence the formation of subsequent questions about the progenitor systems and explosion mecha- generations of stars and the evolution of galaxies. Here we nisms remain. review the observational properties of thermonuclear super- SN Ia are important both to the evolution of the Universe and novae, exploding white dwarf stars resulting from the stellar to our understanding of it. As standardisable candles whose dis- evolution of low-mass stars in close binary systems. The best tance can be observationally inferred8, SN Ia have a starring role known objects in this class are type Ia supernovae (SN Ia), in the discovery of the accelerating expansion of the Universe9, 10 astrophysically important in their application as standardis- and in measurement of its current expansion rate11. SN Ia are able candles to measure cosmological distances and the pri- also major contributors to the chemical enrichment of the Uni- mary source of iron group elements in the Universe. Surpris- verse, producing most of its iron12 and elements nearby in the ingly, given their prominent role, SN Ia progenitor systems periodic table. Because of the stellar evolutionary timescales in- and explosion mechanisms are not fully understood; the ob- volved, the enrichment of these elements occurs differently from servations we describe here provide constraints on models, other elements whose main origin is in massive star supernovae. not always in consistent ways. Recent advances in super- Here we review the observational properties of thermonu- nova discovery and follow-up have shown that the class of clear supernovae, including both normal SN Ia and related ob- thermonuclear supernovae includes more than just SN Ia, jects. We describe the photometric and spectroscopic properties arXiv:1908.02303v1 [astro-ph.HE] 6 Aug 2019 and we characterise that diversity in this review. of SN Ia in section1, and their environments and rates in sec- The modern classification scheme for supernovae traces tion2. Evidence has been growing that not all thermonuclear back to Minkowski1 who in 1941 split “Type I” from “Type explosions of white dwarfs result in “normal” SN Ia; we dis- II” supernovae based on optical spectra. Further subdivision of cuss related supernovae in section3. In this review article we these basic classes has continued on an empirical basis2,3 , and in provide a broad overview supplemented by further discussion of our review we describe the observational properties of what are the newest developments. Our reference list is limited and thus now called SN Ia, along with other similar objects. The obser- necessarily incomplete. We have chosen to highlight illustra- vational classification effort arises from a desire for physical un- tive, recent works with a strong bias towards observations rather than theory or models. These deficiencies are rectified in recent derstanding of these objects, explaining our use of the term ther- 13–15 monuclear supernovae in the title. That categorisation is based reviews that cover many of these topics in more detail . 1 1 Type Ia Supernovae their luminosities by correcting for the duration of the light curve Energetics and light curve properties: The runaway ther- (light curve shape or “stretch”), the optical colour at peak bright- monuclear explosion of a carbon-oxygen white dwarf to iron- ness (“colour”), as well as a correction for the host galaxy prop- group elements releases on the order of 1051 erg as kinetic erties of the SN (see Section2). The original parameterisation energy that unbinds the star. The expanding ejecta travel at of SN Ia light curves was based on the B magnitude decline in ∼10,000 km s−1 and cool rapidly. The luminosity of SN Ia the 15 days after maximum light, ∆m15(B); its correlation with 8 is subsequently powered by the decay of radioactive elements SN Ia luminosity is the Phillips relation . Larger SN Ia samples 32 that were synthesised in the explosion16, 17. The primary power have refined and extended this relation leading to the develop- 33–35 source is the isotope nickel-56, which decays to cobalt-56 with a ment of modern light-curve fitters to derive SN Ia distances . half-life of 6.1 days, and which in turn decays with a half-life of Of particular recent note is the expansion of the wavelength cov- 77.3 days to stable iron-56. The peak SN Ia bolometric luminos- erage of light-curve models to the near infrared, where SN Ia 43 −1 36–39 ity is typically of the order of 10 erg s , with 0.3–0.8 M of appear to be more nearly standard (rather than just standard- iron-56 ultimately produced in each event. The majority (∼85 isable; Figure3) and are less affected by dust extinction. %) of the luminosity of a SN Ia emerges at optical wavelengths SN Ia progenitor systems and explosion mechanisms: Un- and this is where they have been best studied to date. Arnett’s derstanding how and why stellar systems explode to produce rule18 says the peak luminosity of the SN is proportional to the SN Ia is a fundamental astrophysical question and relevant to mass of nickel-56 produced in the explosion, though in general more precise and accurate SN Ia distances for future cosmolog- this is only approximately true19, 20. ical measurements. The research in this area can be divided into The optical light curves of normal SN Ia are relatively ho- two broad categories: studies looking for specific signatures of mogeneous (and can be standardised as discussed below), with the companion star to the primary white dwarf and those that a rise to peak luminosity in ∼20 days, and a slow decline after try to unveil the explosion mechanism that produces the ther- peak before settling on to an exponential decay phase after ∼50 monuclear runaway that unbinds the star. The companion star days (Figure1). The infrared light curves of SN Ia are charac- of SN Ia is thought to be either another degenerate white dwarf terised by a secondary peak 20–30 d after maximum light that is or a non-degenerate star such as a main-sequence, giant, or he- not seen at bluer wavelengths, thought to be driven by the recom- lium star. As such this question is often simplified to asking bination of doubly to singly ionised iron21, 22. SN Ia peak in the whether SN Ia arise from single- or double-degenerate systems. ultraviolet (UV) roughly 15–20 d after explosion, slightly before However, many more questions remain as to how the explosion the optical, but with a much lower flux at most epochs (<10% begins and proceeds: what kind of material is accreted and how of the optical luminosity at peak) due to strong iron-group line quickly? at what mass does the primary white dwarf explode? blanketing opacity. does the explosion start as a subsonic deflagration or a super- Spectral properties of SN Ia: The spectra of SN Ia reveal the sonic detonation? is the primary white dwarf completely dis- elements that are produced in the explosion, their quantities, and rupted or is something left behind? what happens to the com- their location within the SN ejecta (Figure2). SN Ia spectra are panion star? dominated at early times and maximum light by features from Constraining companion stars: Direct searches for compan- intermediate-mass elements such as calcium, magnesium, sil- ion stars to normal SN Ia in data taken either before41 or rela- icon, and sulfur, with typical velocities measured from absorp- tively soon (centuries) after42–45 the explosion have not yielded tion minima of 8000–15000 km s−1 around peak and decreasing any detections. Recently three hypervelocity stars discovered in with time as the photosphere recedes. The earliest spectrum of Gaia data have been proposed as older surviving white dwarf a SN Ia is SN 2011fe at just over one day past explosion25, and companions to SN Ia, arguing for a double-degenerate progeni- it was remarkably similar to maximum-light spectra of SN Ia, tor system without complete disruption of the donor46. apart from the higher velocities.
Details
-
File Typepdf
-
Upload Time-
-
Content LanguagesEnglish
-
Upload UserAnonymous/Not logged-in
-
File Pages14 Page
-
File Size-