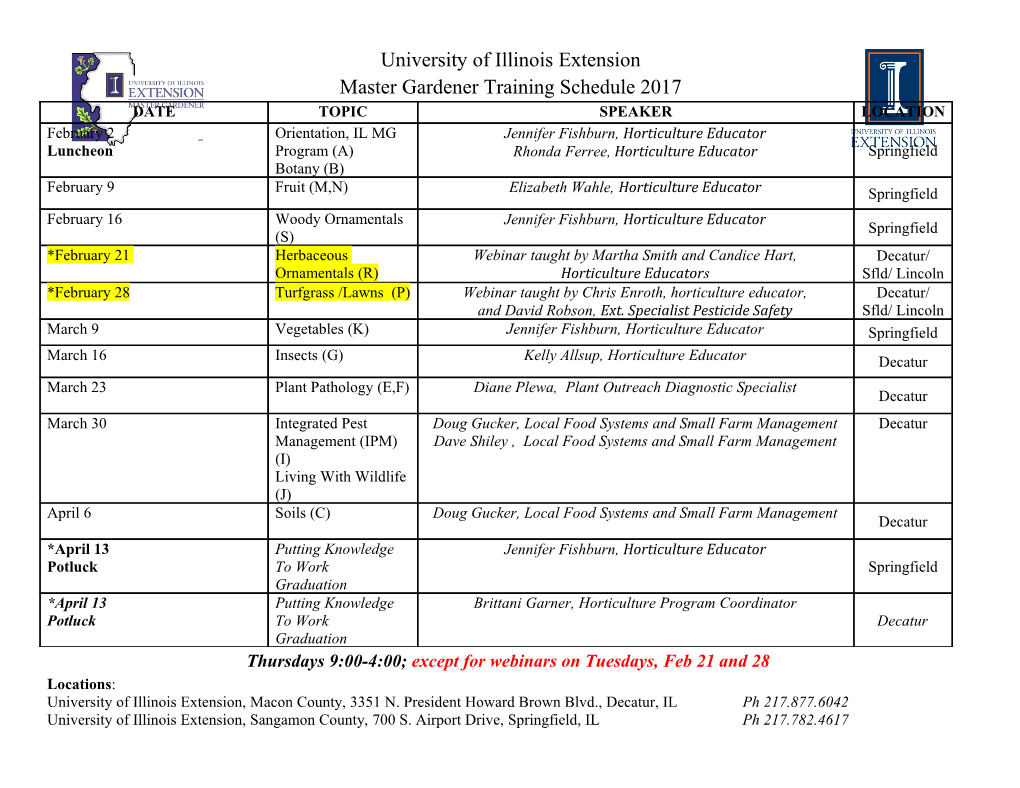
Ocean Sci., 7, 293–304, 2011 www.ocean-sci.net/7/293/2011/ Ocean Science doi:10.5194/os-7-293-2011 © Author(s) 2011. CC Attribution 3.0 License. Flow and mixing near a glacier tongue: a pilot study C. L. Stevens1, C. L. Stewart1, N. J. Robinson1,2, M. J. M. Williams1, and T. G. Haskell3 1National Institute for Water and Atmospheric Research (NIWA), Greta Point Wellington, New Zealand 2University of Otago, Dunedin, New Zealand 3Industrial Research Ltd. (IRL), Gracefield Lower Hutt, New Zealand Received: 2 August 2010 – Published in Ocean Sci. Discuss.: 11 August 2010 Revised: 23 April 2011 – Accepted: 27 April 2011 – Published: 6 May 2011 Abstract. A glacier tongue floating in the coastal ocean the additional frictional boundary effects of the frozen upper presents a significant obstacle to the local flow and so influ- surface (McPhee, 2008). ences oceanic mixing and transport processes. Here acous- Glacier (or ice) tongues add additional complexity to tic Doppler current profiler and shear microstructure obser- coastal ice-ocean interaction. These features, formed by vations very near to a glacier tongue side-wall capture flow glacier outflows into the coastal ocean, can extend many tens accelerations and associated mixing. Flow speeds reached of kilometers from shore (Frezzotti, 1997) and be many hun- around 40 cm s−1, twice that of the ambient tidal flow ampli- dreds of meters thick in places. Glacier tongues and ice tude, and generated vertical velocity shear squared as large shelves significantly influence local circulation and mixing as 10−5 s−2. During the time of maximum flow, turbulent (Jacobs et al., 1981; Legresy et al., 2004). In the case of flow energy dissipation rates reached 10−5 m2 s−3, around three past a glacier tongue, the mixing processes are then a result decades greater than local background levels. This is in keep- of the tidal and regional circulation flows past a bluff body. ing with estimates of the gradient Richardson Number which The closest relevant work is that concerned with flow around dropped to ∼1 during maximum flow. Associated vertical headlands and islands (e.g. Edwards et al., 2004), except of diffusivities estimated from the shear microstructure results course with a glacier tongue the flow can pass under the ob- were substantial, reflecting the influence of the glacier on ve- stacle as well as around. locity gradients. Ice-ocean interaction processes are especially important in southern McMurdo Sound, Antarctica where Haskell Strait connects the western Ross Sea and the single cavity beneath the Ross and McMurdo Ice Shelves (Fig. 1). The Sound 1 Introduction acts as a conduit for both ice shelf waters and warmer Ross Sea waters (Robinson et al., 2010). The fate of these wa- Quantifying ice-ocean interaction, especially at the small- ters is dependent on transport and mixing processes in the scale, is a major challenge in high-latitude earth system sci- region. This exchange influences sea ice growth, which ences (e.g. Hellmer, 2004; Sirevaag et al., 2010; Rignot et in turn affects climate processes over large space and time al., 2010; Dimentrenko et al., 2010; Mahoney et al., 2011) scales (Hellmer, 2004; Dinniman et al., 2007; Robinson et where relatively long timescales and complex thermohaline al., 2010). and pressure effects interact with cryospheric topography The Erebus Glacier Tongue (EGT, Fig. 2) in southern Mc- that is continually changing. Oceanic mixing in polar wa- Murdo Sound has been observed to influence local verti- ters includes different processes relative to those occurring cal stratification through formation of diffusive-convective at lower latitudes. For example, (i) rotational effects are layering (Jacobs et al., 1981). It has also been suggested large, (ii) there is little dynamic influence due to tempera- that such glacier/ice tongues generate local sources of su- ture, (iii) the effect of being close to the freezing temperature percooled water (e.g. Debenham, 1965; Jeffries and Weeks, has a controlling influence on behaviour and (iv) there are 1992) – formed when water is cooled at depth to the in situ freezing temperature but then transported to shallower depths where it is colder than the new in situ freezing tempera- Correspondence to: C. L. Stevens ture. This supercooled water remains liquid until it encoun- ([email protected]) ters nucleation sites at which point it forms frazil/platelet ice Published by Copernicus Publications on behalf of the European Geosciences Union. 294 C. L. Stevens et al.: Flow and mixing near a glacier tongue: a pilot study Fig. 1. Satellite image (SAR) captured on 19 October 2009 showing McMurdo Sound located in the south western Ross Sea connected to the Ross Ice Shelf cavity via Haskell Strait beneath the McMurdo Ice Shelf. The greyscales give an indication of open water and pack ice off Cape Royds and the first year and multiyear ice in the Sound. The inset shows the locale within the western Ross Sea. (e.g. Dmitrenko et al., 2010). Ice crystals grow so large in Fig. 2. ASTER (Advanced Spaceborne Thermal Emission and Re- McMurdo Sound (>250 mm diameter) that the largest of the flection Radiometer) satellite image of south east McMurdo Sound frazil crystals are often referred to as platelets (e.g. Smith et including the Erebus glacier tongue (EGT), the Dellbridge Islands al., 2001). This phenomenon is readily observed in the re- (DI) including Tent Island (TI) and Big Razorback Island (BRI), gion (Dempsey et al., 2010) including during the very first of Erebus Bay (EB), Cape Evans (CE). Cape Armitage (CA), Haskell such measurements by Edward Nelson in 1911 during Scott’s Strait (HS), Scott Base (SB) and the microstructure field camp second expedition (Deacon, 1975). (MSC). The measurements described in Stevens et al. (2006) come The EGT site provides a system in which to examine the from S06. The dashed box shows the locale for Fig. 3a. variability of, and interaction between, small-scale mixing and advective processes next to ice topography. Much work 2 Methods has examined the cryospheric mechanics and the effect of the ocean on the glacier tongue (Robinson and Haskell, 1990; 2.1 Location Squire et al., 1994 and papers therein). Here we present new flow and turbulence measurements in order to quantify the In November 2009 we conducted exploratory oceanographic effect of the glacier tongue on local oceanography. Whilst measurements within 30 m of the sidewall of the EGT at a brief, the sampling was timed such that we captured a di- station called Microstructure Camp (MSC). This was a pilot urnal tidal cycle of turbulence data during the fastest tidal study for a larger experiment to follow. Tidal elevation data flows along with several tidal cycles of upper water column were recorded at Scott Base near Cape Armitage, 17 km to velocity data. These new data are potentially relevant to flow the south (see Fig. 2 for location) and provide a spring-neap and melting processes at the face of larger glacier tongues, context for the experiment. ice shelves and grounded icebergs (Rignot et al., 2010). The objective here is to present new shear microstructure obser- 2.2 Acoustic Doppler current profiler vations providing evidence of substantial ocean mixing rates near the tip of a glacier tongue. The Discussion then explores A 300 kHz acoustic Doppler current profiler (ADCP-RDI the water column structure and kinematics, mixing, mecha- Workhorse) was deployed at the MSC for a four day period nisms for enhancement of local supercooling and general- (Fig. 4), ∼1 km shoreward from the tip on the north side of ization of the results beyond the EGT and how an expanded the EGT (Fig. 3a). The instrument was deployed through a study might extend the present measurements. 650 mm diameter hole in the 2.3 m thick first year fast ice attached to the EGT. The ADCP was mounted just beneath the sea ice at a depth of 2.5 m with the first measurements starting 4 m below this. The ADCP was deployed through Ocean Sci., 7, 293–304, 2011 www.ocean-sci.net/7/293/2011/ C. L. Stevens et al.: Flow and mixing near a glacier tongue: a pilot study 295 Fig. 3. (a) The sampling locale including the microstructure field camp (MSC), around 1000 m east of the tip of the EGT. The black dotted lines highlight the known 100 m contour whilst the blue dashed line shows a tongue of multi year fast ice (i.e. ice connected to the coast or glacier) extending out from the EGT to Big Razor- Fig. 4. The tidal elevation (a) at Scott Base is expanded (b) to high- back Island. (b) A cross-section of the EGT simplified from DeLisle light a four day segment within which our ADCP (grey box in a) et al. (1989). and turbulence profiling (green box in all panels) were recorded. Eastward and northward currents, roughly approximating along and across glacier directions) are shown for depths of (c) 11 and the same hole as the microstructure profiler, with the ADCP (d) 71 m. The grey bar was a period of no data return from the 71 m depth range. being held to one side in a groove. We have experienced substantial interference in similar applications in other ex- periments. However, judicious beam orientation meant there ∂u2 ∂v 2 were no obvious effects in the ADCP data due to the profiler. Sh2 = + . A ∂z ∂z This was established by comparing the individual beams with one another. Also the profiler was actually only beneath the A magnetic declination offset of 144 degrees was included. ADCP for around 50 % of the time and there was no discern- Compass testing did not indicate any inconsistencies due to able difference between the profiler being in the water vs.
Details
-
File Typepdf
-
Upload Time-
-
Content LanguagesEnglish
-
Upload UserAnonymous/Not logged-in
-
File Pages12 Page
-
File Size-