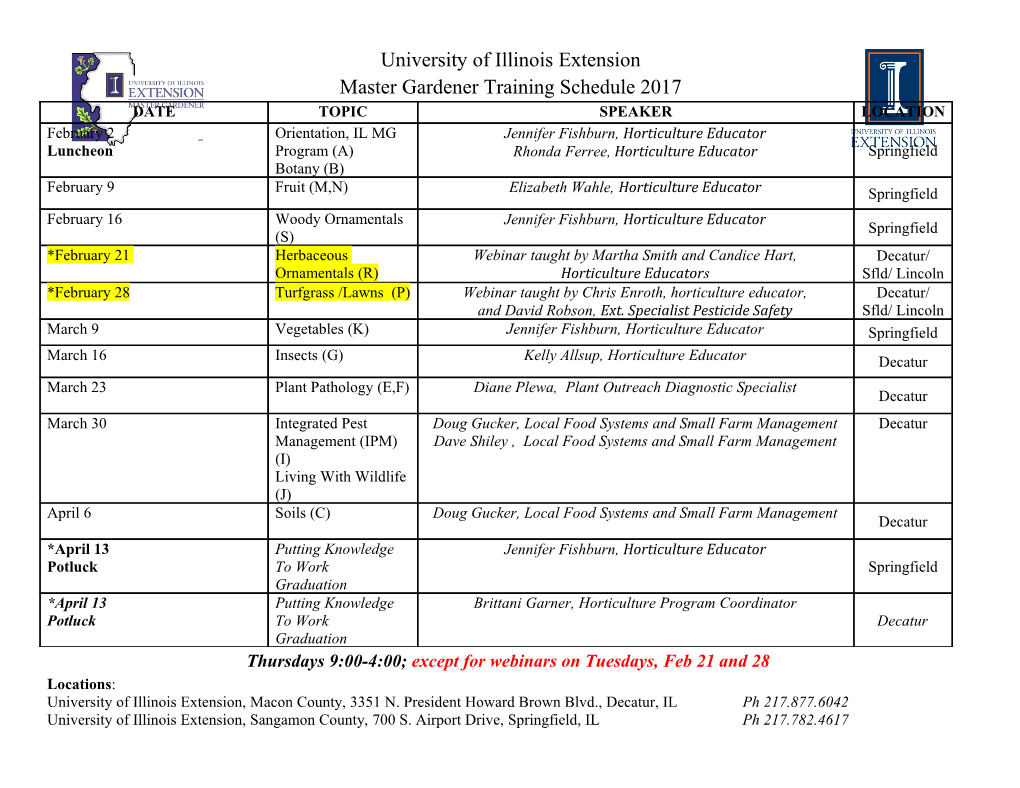
CONSTRAINING SPIRAL GALAXY MASS DISTRIBUTIONS WITH THE RSS IMAGING SPECTROSCOPY NEARBY GALAXY SURVEY By CARL J. MITCHELL A dissertation submitted to the Graduate School|New Brunswick Rutgers, The State University of New Jersey in partial fulfillment of the requirements for the degree of Doctor of Philosophy Graduate Program in Physics and Astronomy written under the direction of J. A. Sellwood and approved by New Brunswick, New Jersey October, 2017 ABSTRACT OF THE DISSERTATION Constraining Spiral Galaxy Mass Distributions with the RSS Imaging Spectroscopy Nearby Galaxy Survey By CARL J. MITCHELL Dissertation Director: J. A. Sellwood Kinematic measurements of galaxies provide a way to trace their mass distributions. The RSS Imaging spectroscopy Nearby Galaxy Survey (RINGS) was designed to probe the mass distributions of 19 spiral galaxies on small spatial scales by obtaining a suite of multi-wavelength kinematic and photometric data. In this dissertation, I introduce the high-spatial-resolution, medium-spectral-resolution Hα kinematic portion of the survey, and detail the process which has been used to convert the raw Fabry-P´erotobservations into useful kinematic data and models. These Hα kinematic data were obtained with the Robert Stobie Spectrograph (RSS) at the Southern African Large Telescope (SALT). I then demon- strate how these Hα kinematic data can be combined with the other types of observations in RINGS, H I radio interferometry and broadband photometric imaging, to probe these galaxies' mass distributions. ii Acknowledgments This work would not have been possible without the contributions of a number of other people. First and foremost, I must thank Jerry Sellwood for providing me with his support and insight over the last several years. I owe considerable gratitude to the other contributors of the RINGS project { Kristine Spekkens and Karen Lee-Waddell, who provided the H I data used in Chapter 4; Rachel Kuzio de Naray and Natasha Urbancic, who provided the photometric data used in Chapter 5; Ted Williams, whose Fabry-P´erotwisdom knows no bounds; and Alex Bixel, who assisted me in the development of my Fabry-P´erotdata reduction code. Conversations with Tad Pryor were especially helpful in understanding the Fabry-P´erot data. Every member of the Rutgers astronomy community has helped me to develop, both as a student and as a person. And of course, this work would not have been possible without the financial support of the NSF, the GAANN program, and the Department of Physics and Astronomy. Finally, I must thank my countless friends and family who have supported me throughout my time here. This research made use of the NASA/IPAC Extragalactic Database (NED) which is operated by the Jet Propulsion Laboratory, California Institute of Technology, under con- tract with NASA; Astropy, a community-developed core Python package for Astronomy (Astropy Collaboration et al. 2013); matplotlib, a Python library for publication quality graphics (Hunter 2007); SciPy (Jones et al. 2001); IRAF, distributed by the National Optical Astronomy Observatory, which is operated by the Association of Universities for Research in Astronomy (AURA) under agreement with the NSF (Tody 1993); and PyRAF, a product of the Space Telescope Science Institute, which is operated by AURA for NASA. Many of the observations described here were obtained with the Southern African Large Telescope. iii Table of Contents Abstract :::::::::::::::::::::::::::::::::::::::::: ii Acknowledgments :::::::::::::::::::::::::::::::::::: iii List of Tables ::::::::::::::::::::::::::::::::::::::: vi List of Figures :::::::::::::::::::::::::::::::::::::: vii 1. Introduction ::::::::::::::::::::::::::::::::::::: 1 1.1. Evidence for Cold Dark Matter . 1 1.2. Cold Dark Matter's Successes and Limitations . 3 1.3. Probing Spiral Galaxy Mass Distributions . 5 1.4. The RSS Imaging-Spectroscopy Nearby Galaxy Survey . 13 2. RINGS Medium-Resolution Hα Fabry-P´erotDataset ::::::::::: 17 2.1. Introduction . 17 2.2. Data Acquisition and Reduction . 20 2.3. Velocity and Intensity Maps . 35 2.4. Summary . 47 3. RINGS Hα Kinematic Modelling :::::::::::::::::::::::: 49 3.1. Introduction . 49 3.2. Axisymmetric Models and Rotation Curves . 50 3.3. Summary . 66 iv 4. NGC 2280 H I Kinematic Data and Comparisons :::::::::::::: 67 4.1. Introduction . 67 4.2. NGC 2280: Basic Properties . 68 4.3. H I Observations . 68 4.4. Velocity Map Comparison . 74 4.5. Modelling Comparison . 77 4.6. Summary . 88 5. NGC 2280 Mass Modeling :::::::::::::::::::::::::::: 90 5.1. Introduction . 90 5.2. Photometric Measurements of NGC 2280's Stellar Mass Distribution . 93 5.3. Determining the Stellar Contribution to the Rotation Curve . 95 5.4. NGC 2280's Dark Matter Halo . 100 5.5. Sources of Uncertainty . 103 5.6. Summary . 107 6. Summary ::::::::::::::::::::::::::::::::::::::: 111 Bibliography ::::::::::::::::::::::::::::::::::::::: 114 v List of Tables 1.1. Galaxies in the RINGS Sample . 14 2.1. RINGS Medium-Resolution Fabry-P´erotObservations . 21 3.1. Best-Fitting Axisymmetric DiskFit Model Parameters . 57 4.1. Galaxies Near NGC 2280 . 72 4.2. NGC 2280 H I Observation Parameters . 73 4.3. NGC 2280 Model Comparison . 78 5.1. Color-Mass-to-Light-Ratio-Relation Model Parameters . 96 vi List of Figures 1.1. Galaxies in the RINGS Sample . 15 2.1. Fabry-P´erotGhosts . 25 2.2. Sample Fabry-P´erotspectra and line profile fits . 32 2.3. NGC 337A Fabry-P´erotData . 35 2.4. NGC 578 Fabry-P´erotData . 36 2.5. NGC 908 Fabry-P´erotData . 36 2.6. NGC 1325 Fabry-P´erotData . 37 2.7. NGC 1964 Fabry-P´erotData . 37 2.8. NGC 2280 Fabry-P´erotData . 38 2.9. NGC 3705 Fabry-P´erotData . 38 2.10. NGC 4517A Fabry-P´erotData . 39 2.11. NGC 4939 Fabry-P´erotData . 39 2.12. NGC 5364 Fabry-P´erotData . 40 2.13. NGC 6118 Fabry-P´erotData . 40 2.14. NGC 6384 Fabry-P´erotData . 41 2.15. NGC 7606 Fabry-P´erotData . 41 2.16. NGC 7793 Fabry-P´erotData . 42 2.17. Radial R-band Surface Brightness Profiles . 44 2.18. Radial Hα Intensity Profiles . 45 2.19. Radial N2 Index Profiles . 46 2.20. Radial Oxygen Abundance Profiles . 47 vii 3.1. NGC 337A DiskFit Axisymmetric Model . 52 3.2. NGC 578 DiskFit Axisymmetric Model . 52 3.3. NGC 908 DiskFit Axisymmetric Model . 53 3.4. NGC 1325 DiskFit Axisymmetric Model . 53 3.5. NGC 1964 DiskFit Axisymmetric Model . 53 3.6. NGC 2280 DiskFit Axisymmetric Model . 54 3.7. NGC 3705 DiskFit Axisymmetric Model . 54 3.8. NGC 4517A DiskFit Axisymmetric Model . 54 3.9. NGC 4939 DiskFit Axisymmetric Model . 55 3.10. NGC 5364 DiskFit Axisymmetric Model . 55 3.11. NGC 6118 DiskFit Axisymmetric Model . 55 3.12. NGC 6384 DiskFit Axisymmetric Model . 56 3.13. NGC 7606 DiskFit Axisymmetric Model . 56 3.14. NGC 7793 DiskFit Axisymmetric Model . 56 3.15. Rotation Curve Literature Comparison . 59 4.1. R-band, Hα, and H I Intensity Maps of NGC 2280 . 69 4.2. NGC 2280 Line Profile Fits . 70 4.3. NGC 2280 Hα Fabry-P´erotKinematic Map . 71 4.4. NGC 2280 H I Kinematic Map . 75 4.5. NGC 2280 Velocity Difference Map . 77 4.6. NGC 2280 Model Comparison . 79 4.7. NGC 2280 Model Residuals . 80 4.8. NGC 2280 Hα and H I Rotation Curve Comparison . 82 4.9. NGC 2280 Rotation Curve Comparison - Approaching/Receding . 83 4.10. NGC 2280 Anomalous H I Line Profiles . 85 4.11. NGC 2280 Beam-Smeared Rotation Curves . 86 viii 4.12. NGC 2280 Hα and H I Intensity Profiles . 87 5.1. NGC 2280 BV Photometry . 94 5.2. NGC 2280 Stellar Mass Maps . 97 5.3. NGC 2280 Exponential Disk Fits . 99 5.4. NGC 2280 Stellar Rotation Curve Contributions . 101 5.5. NGC 2280 Halo Density Profiles . 102 5.6. NGC 2280 Halo Profile Functional Fits . 104 5.7. Effects of CMLR Normalization Uncertainty . 105 5.8. Effects of Distance Uncertainty . 106 5.9. Effects of Vertical Profile Uncertainty . 108 ix 1 Chapter 1 Introduction The formation and evolution of galaxies are well-explained by the so-called ΛCDM paradigm. In ΛCDM, the energy content of our universe is dominated by a repulsive energy density dubbed \dark energy" and an invisible matter component known as \dark matter." Only about 5% of the universe is composed of the ordinary matter with which we are most familiar (Komatsu et al. 2009). ΛCDM has been extremely successful as a theory, explaining a wide range of astro- nomical phenomena across a wide range of spatial scales (see review by Somerville & Dav´e 2015). Despite this, there remain some open questions, particularly on small scales within the central regions of galaxies. In this introduction, I provide an overview for the evidence for the \CDM" in ΛCDM, the \Cold Dark Matter," and discuss some of its successes and current limitations as a theory, in particular the difficulty of testing it on small spatial scales. I then briefly discuss some of the wide range of methods that can be used to probe the mass distributions of galaxies. Finally, I introduce the RSS Imaging-Spectroscopy Nearby Galaxy Survey (RINGS), which was designed to utilize some of these techniques to probe galaxy mass distributions on small spatial scales. 1.1 Evidence for Cold Dark Matter Galactic rotation curves were one of the earliest probes of galaxy mass distributions and provide one of the most stark pieces of evidence for the existence of dark matter. In spiral 2 galaxies, stars and gas rotate in a plane. A rotation curve is a measurement of the speed of this rotation as a function of radius, i.e. Vc(R), where Vc is the circular speed and R is the 2-dimensional radius measured in the plane of the disk (e.g. Rubin et al. 1982). In these galaxies, the light coming from stars is known to roughly follow a distribution that declines exponentially with radius, i.e.
Details
-
File Typepdf
-
Upload Time-
-
Content LanguagesEnglish
-
Upload UserAnonymous/Not logged-in
-
File Pages130 Page
-
File Size-