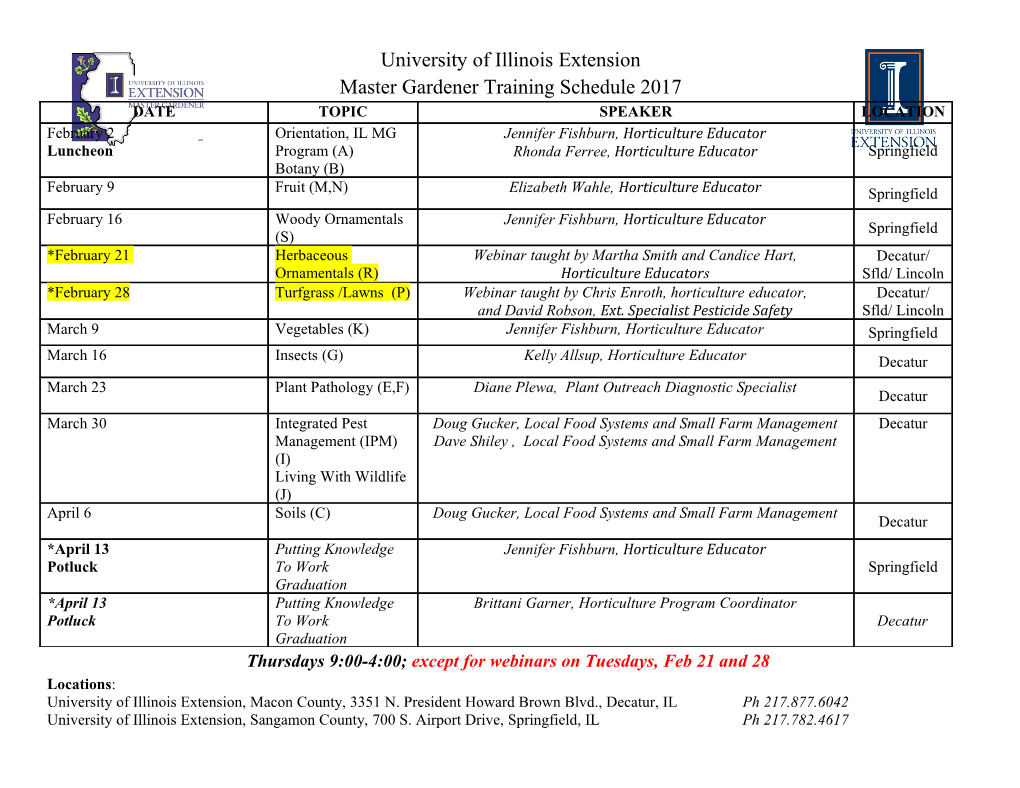
nutrients Article Saccharin and Sucralose Protect the Glomerular Microvasculature In Vitro against VEGF-Induced Permeability Emmanuella Enuwosa 1, Lata Gautam 2, Linda King 1 and Havovi Chichger 1,* 1 Biomedical Research Group, Faculty of Science & Engineering, School of Life Sciences, Anglia Ruskin University, Cambridge CB1 1PT, UK; [email protected] (E.E.); [email protected] (L.K.) 2 Forensic Science Research Group, Faculty of Science & Engineering, School of Life Sciences, Anglia Ruskin University, Cambridge CB1 1PT, UK; [email protected] * Correspondence: [email protected] Abstract: Diabetic kidney disease (DKD) has become a global health concern, with about 40% of people living with type 1 and type 2 diabetes mellitus developing DKD. Upregulation of vascular endothelial growth factor (VEGF) in the kidney is a significant pathology of DKD associated with increased glomerular vascular permeability. To date, however, current anti-VEGF therapies have demonstrated limited success in treating DKD. Recent studies have shown that artificial sweeteners exhibit anti-VEGF potential. The aim of this study was therefore to assess the effects of aspartame, sac- charin, and sucralose on VEGF-induced leak using an in vitro model of the glomerular endothelium. Saccharin and sucralose but not aspartame protected against VEGF-induced permeability. Whilst the sweeteners had no effect on traditional VEGF signalling, GC-MS analysis demonstrated that the sweetener sucralose was not able to enter the glomerular endothelial cell to exert the protective effect. Chemical and molecular inhibition studies demonstrated that sweetener-mediated protection of the glomerular endothelium against VEGF is dependent on the sweet taste receptor, T1R3. These studies Citation: Enuwosa, E.; Gautam, L.; King, L.; Chichger, H. Saccharin and demonstrate the potential for sweeteners to exert a protective effect against VEGF-induced increased Sucralose Protect the Glomerular permeability to maintain a healthy endothelium and protect against vascular leak in the glomerulus Microvasculature In Vitro against in settings of DKD. VEGF-Induced Permeability. Nutrients 2021, 13, 2746. https:// Keywords: artificial sweeteners; diabetic kidney disease; sweet taste receptor; glomerular; doi.org/10.3390/nu13082746 endothelium; vascular endothelial growth factor (VEGF); gas chromatograph-mass spectrometry (GC-MS); vascular permeability Academic Editors: Vasanti S. Malik and Valisa Hedrick Received: 22 June 2021 1. Introduction Accepted: 6 August 2021 Published: 10 August 2021 The increasing incidence of diseases linked to higher consumption of sugar-sweetened drinks has led to a shift towards a supposedly healthier option, such as low-calorie and Publisher’s Note: MDPI stays neutral non-nutritive sweeteners. Amongst these sweeteners are both synthetic (artificial) and with regard to jurisdictional claims in naturally derived sweet-taste molecules, of which artificial sweeteners are more commonly published maps and institutional affil- found in both low-calorie and sugar-free foods and drinks [1]. The most commonly used, ® iations. commercially available artificial sweeteners are acesulfame–K (Sunnett , New York, NY, USA), aspartame (NutraSweet®, Augusta, GA, USA), saccharin (Sweet’N Low®, New York, NY, USA), and sucralose (Splenda®, Carmel, CA, USA) [2]. These sweeteners vary in their structure, intensity of sweetness, after-taste, and pharmacokinetics [1]. Artificial sweeteners elicit a distinctive perceived quality—termed “sweetness”—in humans through binding to Copyright: © 2021 by the authors. Licensee MDPI, Basel, Switzerland. the sweet taste receptor [3]. In humans, the sweet taste receptor belongs to the T1R class This article is an open access article C of G protein-coupled receptors (GPCRs). The T1R has two subtypes: the T1R member distributed under the terms and 2 and 3 (T1R2 and T1R3), which form a heterodimer to act as a sweet taste receptor [4]. conditions of the Creative Commons Whilst the T1R2/T1R3 is a heterodimer, the T1R3 subunit can also act as a homodimer to Attribution (CC BY) license (https:// form a sweet taste sensor [3,5]. Indeed, the T1R3 subunit alone has been shown to play creativecommons.org/licenses/by/ a vital role in sweet taste sensing in the vasculature [6–8]. The sweet taste receptor acts 4.0/). through the traditional GPCR-signalling pathway to activate the βγ G-protein subunits and Nutrients 2021, 13, 2746. https://doi.org/10.3390/nu13082746 https://www.mdpi.com/journal/nutrients Nutrients 2021, 13, 2746 2 of 16 phospholipase C-β (PLC-β) to stimulate cAMP production, triggering intracellular calcium release and resulting in sweet taste perception in the sweet taste buds in the mouth [9–11]. Of significance, the sweet taste receptor has been identified in a range of extraoral locations including the small intestine, heart, bladder, and bone [6,12–14]. Recently, the sweet taste receptor has also been identified in the vasculature of the lungs and retina, where it plays a key role in regulating endothelial barrier function and attenuating aberrant angiogenic processes [7,8]. These findings raise the question of whether the expression of sweet taste receptors occurs in other vascular beds and their potential impact on mechanisms related to vascular disruption. Diabetic kidney disease (DKD) is a form of microvascular complication and the major cause of end-stage renal disease in patients with diabetes [15–17]. The growing number of people with diabetes has a direct impact on DKD incidence, with approximately 30% and 40% of individuals with type 1 (T1DM) and type 2 (T2DM) diabetes mellitus respectively developing DKD over a median of 15 years from the time of disease diagnosis [18–21]. DKD is a progressive condition that begins with the thickening of the glomerular basement membrane, associated with loss of endothelial fenestrations and podocytes, followed by de- velopment of microaneurysms, leading to exudative lesions from subendothelial deposits of plasma proteins [22–26]. In later stages of DKD, interstitial changes and glomerulopathy can result in segmental and global sclerosis, associated with albuminuria [17]. Studies have shown that in DKD patients and rodent models of the disease, an increase in vascular endothelial growth factor (VEGF) has been associated with increased vascular permeability linked to hyperfiltration and albuminuria [27–30]. Given the pivotal role of glomerular vascular permeability in regulating DKD pathology, therapies for the disease have been focused on anti-VEGF approaches to attenuate this leak. Whilst effective in rodent mod- els, a subset of diabetic patients treated with anti-VEGFs, such as Bevacizumab, display worsening of renal injury and excessive albuminuria [31,32]. There is, therefore, a need to develop vascular-specific therapeutics to reduce vascular permeability and therefore albuminuria in patients living with DKD without further exacerbating the disease. In the present study, our central hypothesis was that artificial sweeteners can protect the glomerular endothelium against VEGF-induced permeability. We proposed that this protective effect could be mediated by the sweet taste receptor, T1R3. We further sought to establish the molecular mechanism through which artificial sweeteners exert this protective effect, with a focus on cAMP-dependent signalling and oxidative stress in the endothe- lium. This study aimed to expand our understanding of the effect of sweeteners on the vasculature and potentially identify a new therapeutic option in the treatment of vascular disruption in DKD. 2. Materials and Methods 2.1. Cell Line and Reagents Primary glomerular microvascular endothelial cells (GMVEC), purchased from Cell Systems (Kirkland, WA, USA), were cultured in complete classic medium (#4Z0-500) sup- plemented with culture boost. The passage number was used between 3 to 7. This primary cell line was utilised as any other relevant cell lines are immortalised rather than human primary cells and were therefore not appropriate. Endothelial growth factor (VEGF-A165) was purchased from Thermo-Fisher (Paisley, UK). Pure and analytical grade artificial sweeteners, aspartame, saccharin, and sucralose, the CCK-8 cell viability kit, FITC-dextran, N-Methyl-N-(trimethylsilyl) trifluoroacetamide (MSTFA), methanol, and ethyl acetate were purchased from Sigma-Aldrich (Dorset, UK). Lactisole, a sweet taste inhibitor, was pur- chased from Cayman Chemical (Ann Arbor, MI, USA). The cAMP-Screen Direct System kit and GSH Bioxytech activity kit were purchased from Applied Biosystems and Merck Milli- pore respectively. DharmaFECT™ reagent and siRNA (T1R3 and non-specific, scrambled) were purchased from Dharmacon (Cambridge, UK). Anti VE-cadherin and fluorescent secondary antibodies, deuterated sucralose, and sucralose-D6 (SC-220145) were purchased Nutrients 2021, 13, 2746 3 of 16 from Santa Cruz Biotechnology (Santa Cruz, CA, USA). 2,7-dichlorodihydrofluorescein diacetate (DCFDA) was purchased from Abcam (Cambridge, UK). 2.2. Cell Viability Assay The effect of the artificial sweeteners on GMVEC viability was determined using the cell-counting kit-8 (CCK-8), following manufacturer’s guidelines. GMVEC were exposed to increasing concentrations (0.1–100 µM) of aspartame, saccharin, and sucralose separately and incubated for 24 h. A vehicle control of H2O was used for 0 µM. Absorbance was measured at 450 nm using a multi-mode microplate reader (Tecan Sunrise), and viability was calculated as % normalised to vehicle. 2.3. Endothelial
Details
-
File Typepdf
-
Upload Time-
-
Content LanguagesEnglish
-
Upload UserAnonymous/Not logged-in
-
File Pages16 Page
-
File Size-