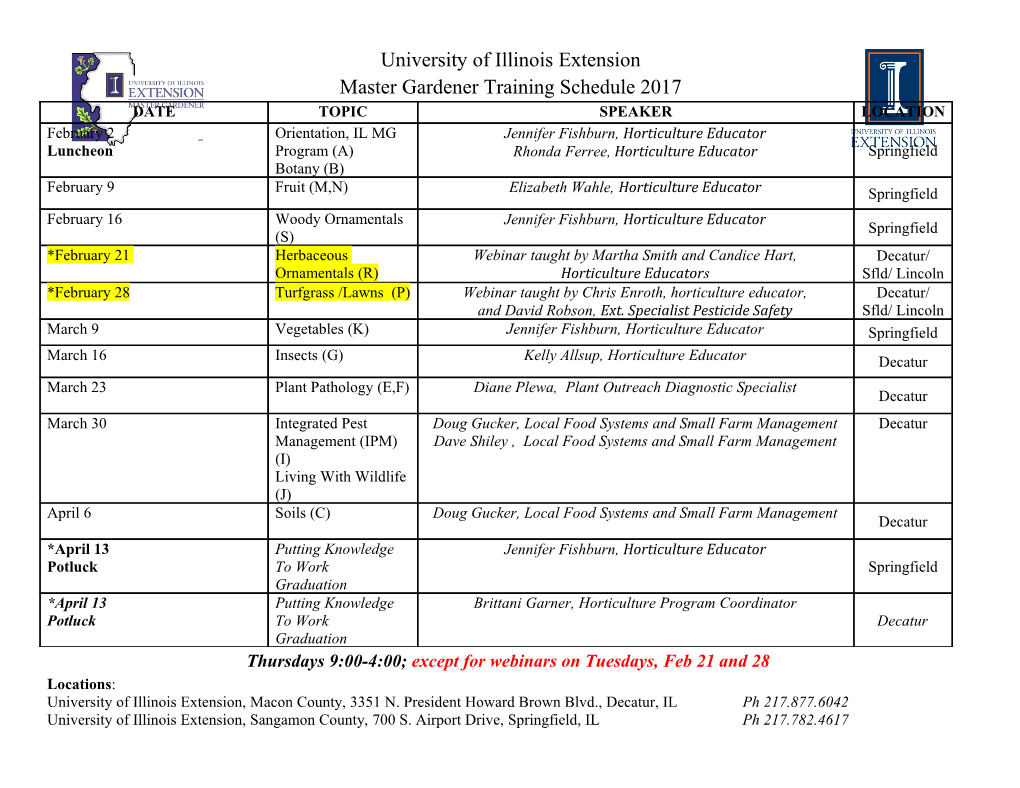
8th IAHR ISHS 2020 Santiago, Chile, May 12th to 15th 2020 DOI: 10.14264/uql.2020.517 Towards a multi-hazard analysis of infrastructure in a seismic coast subjected to climate change, with a focus on the Chilean coastline P. Winckler1 1Escuela de Ingeniería Civil Oceánica Universidad de Valparaíso Centro de Observación Marino para estudios de Riesgos del Ambiente Costero (COSTAR) Centro Nacional de Investigación para la Gestión Integrada de Desastres Naturales (CIGIDEN) Valparaíso, Chile E-mail: [email protected] ABSTRACT The physics of some of the most relevant climatic and geophysical drivers affecting the Chilean coasts are reviewed. Some relevant figures about the country are introduced, and recent findings and research gaps on extreme waves, meteotsunamis, storm surge, sea level rise, earthquakes and tsunamis along the country are analyzed. A discussion on how to combine these phenomena to assess the level of flooding from a multi-hazard perspective is proposed, and a conceptual framework for the integration of short-term meteorological effects, long-term climate driven hazards and geophysical drivers into a multi-hazard analysis is discussed. Finally, the identification of gaps and future research is proposed. The multi-hazard, probabilistic and nonstationary design approach proposed herein, where uncertainty is at stake, can be extended to the design of coastal infrastructure, such as the stability of breakwaters, overtopping and forces on slender elements. Keywords: Multi-hazard analysis, coastal flooding, climate change 1. INTRODUCTION The physical impacts in the coastal territory stemming from a combination of short-term meteorological effects (coastal storms, meteotsunamis and storm surge), long-term climate driven hazards (sea-level rise) and astronomical tides include, among others, flooding of coastal areas; erosion of beaches, cliffs, river outlets and dunes; changes in the dynamics of wetlands and estuaries; increased downtime and operational delays in commercial and minor ports and damage to coastal infrastructure. These climate driven impacts can be enhanced by other fast-occurring geophysical hazards (earthquakes, tsunamis), slow seafloor changes of anthropogenic source (e.g. subsidence due to the compaction of sediment by buildings or groundwater extraction) or shortage of sediment supply during the design life of a structure. In the most general sense, the flooding level with respect to a present-day lowest astronomical tide (LAT) can be computed as ∆ , 1 where is the astronomical tide, ∆ is the sea-level rise between a reference and a target period, is the storm surge, is the meteotsunami, is the wave setup, is the wave runup, is the tsunami runup and considers ingragravity waves and other effects, such as river flooding. Naturally, these variables occur at very different spatial and time scales and with very different amplitudes depending on the local conditions of a site. Equation (1) is based on a simple additive model where variables are assumed independent and nonlinear interactions among them are disregarded. It can either be understood from both the deterministic or probabilistic points of view (e.g. using Monte Carlo simulations), requiring in both cases long term records for each variable and correlations to understand their dependency. Additionally, it can be computed from a traditional approach based on historical data, or considering climate projections (Toimil et al. 2019). From an applied standpoint, coastal structures in seismic-prone regions are usually designed by separating short and long-term climate driven variables (Figure 1) from earthquakes and tsunamis. Under this simplification, Equation (1) reduces to ∆ , (2) where waves and sea level are obtained from hindcasts (e.g. Beyá et al 2017; Winckler et al, 2019) or from Global Climate Models (GCMs) in case climate change is considered. If only geophysical phenomena are considered, Equation (1) becomes: , (3) where the coseismic uplift/subsidence is considered as a change in the LAT (Figure 2). In Equations (2) or (3), the astronomical tide are usually included as a static level above the LAT. Figure 1: Climate-driven and astronomical tide used to define the flooding level. During the design life of a structure, a combination of short and long-term climate with geophysical phenomena can occur, thus altering the expected risks and possible economic consequences of failure. Despite this, there are few studies proposing methodologies to combine short-term meteorological effects and long-term climate driven hazards in the design of coastal structures (e.g. Vousdoukas et al. 2017) and even less studies combining tsunami flooding with sea level rise (e.g. Li et al. 2018). The long-term projection and combination of climate-driven hazards, on the other hand, is relatively novel. Mori et al. (2016), for example, combined sea level rise, storm surge and extreme waves to evaluate the influence of future climate change on caisson breakwaters while Lee et al. (2013) conducted a reliability analysis for wave run-up and armor stability of inclined coastal structures for various scenarios of long-term sea level rise due to climate change. To the author’s level of knowledge, which is obviously limited, no comprehensive studies have been conducted to include all variables in Equation (1) in the context of risk analysis of coastal structures. Figure 2: Coseismic seafloor changes, tsunamis and astronomical tide used to define the flooding level. 2. THE LOCAL CONTEXT Chile has an extensive coastline of ~4200 km facing the southeast Pacific Ocean (Figure 3A). The territory is characterized by strong latitudinal gradients, with a climate ranging from the most arid desert worldwide in the north to rainforests in Patagonia. Its geomorphology is shaped by the Chile-Peru trench, formed by the subduction of the Nazca Plate beneath South America. Between 18.4°S and 41.5°S, an almost rectilinear coastline is comprised by cliffs, dune fields, coastal wetlands, peninsulas and few bays where major ports cities are situated. This coastline has one of the narrowest continental shelves worldwide (Paris et al. 2016) showing an intense seismicity and tsunami generation potential. In contrast, the south (41.5°S to 55.6°) represent the most extensive fjord region in the world (Cameron and Pritchard 1963). These features provide unique atmospheric, oceanographic and geologic conditions which make the country a natural laboratory to investigate climate trends and coastal hazards, with similarities with countries found the Pacific coasts of South, Central and North America, Alaska, Japan and Indonesia. Indeed, between 1980 and 2011, Chile recorded losses of nearly 1.2% of its GDP due to natural disasters, a significant part of which is due to meteorological drivers (floods, winds, and storm surge), earthquakes and tsunamis (UNISDR 2015). Figure 3B depicts the offshore mean wave climate while Figure 3C shows the population in coastal municipalities. Winckler et al. (2019) indicates that nearly 972 thousand inhabitants lived in less than 10 meters above sea level in 2017, figure which is lower than the global and Latin American average, with 10% and 6%, respectively (McGranahan et al. 2007). According to Church et al. (2013), the rapid increase in coastal population will boost the risk of climate related disasters. A) B) C) Figure 3: A) Typical atmospheric pressure pattern including the Southeast Pacific Anticyclone and an westward extratropical cyclone L (orange), tectonic setting (pink) and tide gauges with records of more (blue triangles) or less (red dots) than 30 years of data. B) Mean wave direction and significant wave height () offshore Chile (adapted from Beyá et al. 2017). C) Population of coastal municipalities, according to 5 censuses (INE 2017). 3. MULTI-HAZARDS 3.1. Extreme waves Wave climate is controlled by swells emerging from westward extratropical cyclones (Figure 3A) with trajectories over almost 2000 km, following a latitudinal belt between 40°S and 60°S (Beyá et al. 2017). The intense surface winds associated with extratropical cyclones transfer energy to waves, which propagate from the South Pacific Ocean towards South American coasts. Due to Chile’s length, there are strong latitudinal gradients in mean wave climate (Figure 3B), ranging from highly energetic western swells in the south to a relatively mild wave climate in the far north due to the greater distance from the wave generation zone. A) B) Figure 4: A) Number and B) Rate of change of extreme wave events along the Chilean coast, based on a wave hindcast (Beyá et al., 2017) for the period 1980-2015 (Winckler et al. 2019). Based on a wave hindcast (Beyá et al., 2017) for the period 1980-2015, Winckler et al. (2019) shows a significant increase in the number extreme wave climate throughout the entire coastal zone (Figure 4), while the number of extreme wave events increased between 4 to 12 events since the early 80’s in the northern and southernmost extremes of the country, respectively. As for the future, the frequency and intensity of extreme waves is expected to increase by 2045 in the main 9 ports which are exposed to the Pacific Ocean (Figure 5). A) B) C) D) Figure 5: Projected extreme values of computed as the median of six wave models in 9 ports (Winckler et al 2019). Thick circles correspond to the analysis in Valparaíso. A) Historical period (1985-2004), B) projection (2026-2045), C) absolute difference and D) relative difference between both periods are presented. 3.2. Meteotsunamis and storm surges Meteotsunamis and storm surges are long waves caused by storms, the former usually ranging between 5 minutes and 2 hours and the later between 2 hours and days. Meteotsunamis seldom occur along the west coast of South America and, when combined with other oceanographic conditions (extreme waves and storm surge), may cause damage levels comparable to those resulting from Mw 8 earthquake generated tsunamis (Carvajal et al., 2017). Storm surges are relatively minor along the Chilean coasts basically due to the presence of the Perú-Chile trench and the relatively small continental shelf (Paris et al.
Details
-
File Typepdf
-
Upload Time-
-
Content LanguagesEnglish
-
Upload UserAnonymous/Not logged-in
-
File Pages14 Page
-
File Size-