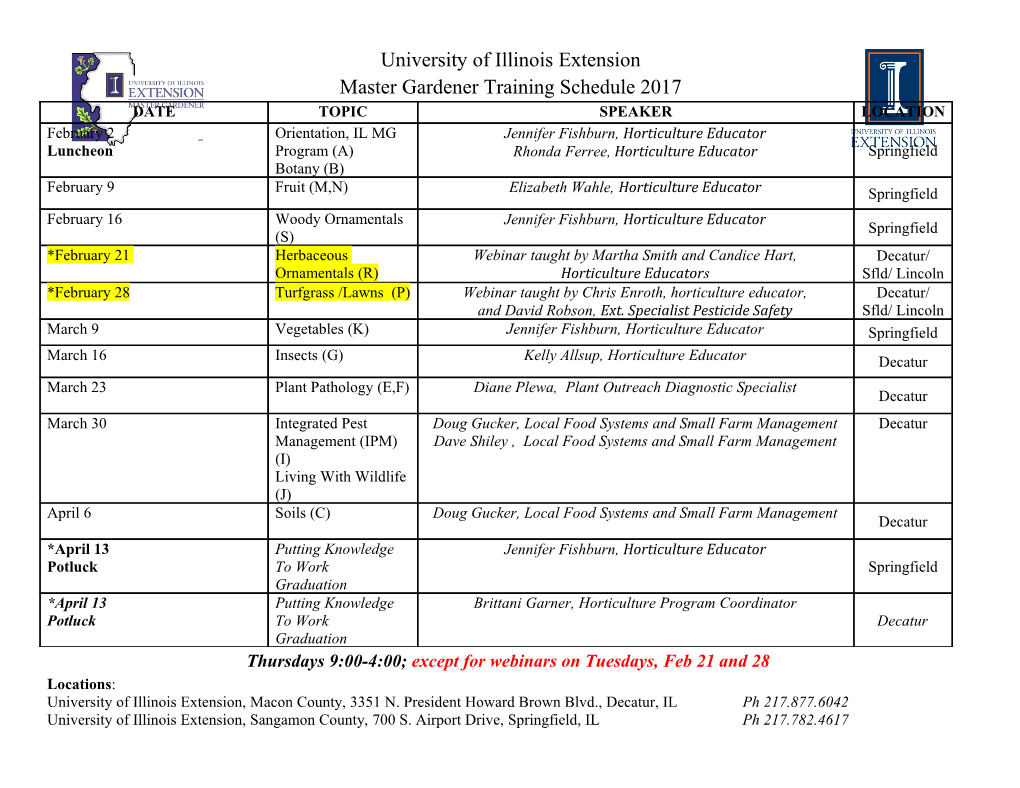
SCHUBERT CALCULUS AND QUIVER VARIETIES ALLEN KNUTSON CONTENTS Introduction 2 Notational conventions 2 Part 1. Equivariant Schubert calculus 2 1. Flag and Schubert varieties 2 1.1. Atlases on flag manifolds 3 1.2. The Bruhat decomposition of Gr k; Cn 4 1.3. First examples of Schubert calculus 6 1.4. The Bruhat decomposition of flag( manifolds) 7 1.5. Poincare´ polynomials of flag manifolds 8 1.6. Self-duality of the Schubert basis 9 1.7. Push and pull operations 10 1.8. Borel’s presentation of ordinary cohomology: stable case 12 1.9. Borel’s presentation of ordinary cohomology: unstable case 15 1.10. Divided difference operators and Schubert polynomials 17 1.11. Kleiman’s theorem. Examples of Schubert calculus. 19 2. A first look at puzzles 20 2.1. Grassmannian puzzles 20 2.2. Rotational symmetry of puzzles 22 2.3. Separated-codescent puzzles 23 2.4. Inventing 2-step puzzles. 24 3. Equivariant cohomology 25 3.1. Recalling ordinary cohomology 25 3.2. Kernels and convolution 26 3.3. Equivariant cohomology: properties and definition 28 3.4. From G to T. 31 3.5. Convolution in equivariant cohomology 32 4. Equivariant cohomology of the flag manifold 35 4.1. The equivariant Borel presentation 35 4.2. Double Schubert polynomials 35 4.3. Push-pull vs. sweeping 36 4.4. Point restrictions of Schubert classes 38 4.5. The AJS/Billey formula, in terms of Rˇ-matrices 42 Date: October 28, 2019. 1 2 ALLEN KNUTSON 5. Equivariant separated-descent puzzles. Proof via YBE. 42 (1) (2) Part II: Quiver varieties. (a) Definition. Deformation to affine. Cotangent bundles. (b) Nakajima’s theorem on Htop. Nakajima’s reflection isomorphisms. (c) The “category” of symplectic reductions. Examples. (d) Maulik-Okounkov stable envelopes, via the deformation to affine. (e) Maulik-Okounkov bases and R-matrices. (f) Varagnolo’s theorem: the Yangian action on H∗. (3) Part III: Schubert calculus and quiver varieties. (a) Segre-Schwartz-MacPherson classes on Grassmannians, and puzzles. (b) Proof via symplectic reductions. (c) Separated-descent puzzles and their proof. (d) Associativity. (e) d 2; 3; 4. (4) Part IV: Complements. (a) Noncommutative= deformation and Verma modules. (b) Chern-Schwartz-MacPherson classes. (c) Self-dual puzzles. (d) Equivariant K-theory. (e) Rigid puzzles and synthetic solutions. (f) Belkale-Kumar puzzles. (g) The origin of puzzles in studying sums of Hermitian matrices. Introduction NOTATIONAL CONVENTIONS Part 1. Equivariant Schubert calculus 1. FLAG AND SCHUBERT VARIETIES Let V denote an n-dimensional vector space over C, and 0 n1 n2 ::: nd n a sequence of d integral “steps”. Our principal object of study in this book is the d-step flag variety ≤ ≤ ≤ ≤ ≤ Fl n1; n2; : : : ; nd; V V1;V2;:::;Vd Vi Vi+1 V; dim Vi ni; i 1; : : : ; d So far( this is a set, of “d)-step∶= ( flags of subspaces) ∶ in≤ V”;≤ our first endeavor= ∀ is= to give it the structure of complex manifold (or more specifically, smooth complex variety). When V Cn, there is a natural map n = rowspan GLn C Fl n1; n2; : : : ; nd; C M :::; span of top ni rows, ::: i 1:::d ∶ ( ) → ( ) = ↦ ( ) SCHUBERT CALCULUS AND QUIVER VARIETIES 3 which is easily seen to be onto (pick a basis of V1, extend to a basis of V2, . , extend to a basis of Cn, take that basis as rows of M). This map rowspan is equivariant with respect 1 to the right action of GLn C , transitive on both spaces. The basic case d 1 gets a special name and notation; it is the Grassmannian Gr n1; V Fl n1; V of n1-planes in V( (and) we will usually write k instead of n1). We consider this case first, to later build= on it through the obvious inclusion ( ) ∶= ( ) d Fl n1; n2; : : : ; nd; V Gr ni; V i=1 ( V1;V2;:::;Vd) ↪ MV1;V(2;:::;V)d whose image is the nested tuples, satisfying V1 V2 ::: Vd. ( ) ↦ ( ) The full flag variety Fl 1; 2; : : : ; n; Cn also gets special notation, Fl Cn . ≤ ≤ ≤ 1.1. Atlases on flag manifolds.( To make) Gr k; V into a complex manifold,( ) not just a set, we give it an atlas of charts whose transition maps are holomorphic (in fact, algebraic). Begin by defining the Stiefel manifold ( ) n Stiefel k; C k n complex matrices of rank k and consider the surjective map ( ) ∶= { × } n n rowspan Stiefel k; C Gr k; C n The Stiefel manifold is a Zariski open set inside Mk n, the union of principal open sets ∶ ( ) ×↠ ( ) k n Uλ M Mk n det columns λ in M 0 λ × k [ ] n The fibers of the map∶= {rowspan∈ are∶ exactly( the left GL)k ≠C }-orbits∈ on Stiefel k; C , and this action preserves each of the open sets Uλ. Conveniently, this action on Uλ admits a continuous system of orbit representatives: ( ) ( ) ′ Uλ M Mk×n columns λ in M form an identity matrix and consequently ∶= { ∈ ∶ } n rowspan ′ U′ Gr k; the restriction Uλ λ C is injective, and n the images rowspan U′ , λ [ ] cover Gr k; Cn ● λS ∶ k→ ( ) if we give Gr k; Cn the quotient topology, i.e. the coarsest topology such that ● rowspan is continuous( ) (w.r.t.∈ the Zariski( topology) on Stiefel k; Cn ), then the ● ( ′) [n] images rowspan Uλ , λ k are open. ( ) n n Theorem 1. The charts rowspan U′ U′ Gr k; form an algebraic atlas, making Gr k; ( ) ∈ λ λ C C a smooth scheme over C of dimension k n k . S ∶ → ( ) ( ) Proof. G rowspan U′ rowspan U′ G rowspan U rowspan U Let λµ λ ( − ) µ , so λµ λ µ ′ ′ rowspan Uλ Uµ . Its preimages in Uλ and Uµ are respectively ′ = ( ) ∩ ( ) = ( ) ∩ ( ) = U Uµ M Mk n columns λ in M form an identity matrix, det columns µ 0 λ ( ∩ ) × and the very similar U′ Uλ. The overlap map U′ Uµ Gλµ U′ Uλ is ∩ = { ∈ µ ∶ λ µ ( ) ≠ } M µ M −1M ∩ columns in ∩ →̃ →̃ ∩ which is algebraic. ↦ ( ) 1 n For a number of reasons we choose C to, as a rule, denote row vectors. One important one is that wide, shallow matrices fit better on the page. 4 ALLEN KNUTSON ′ 2 As to the dimension, the conditions on each Uλ specify k of the kn matrix entries, leaving k n k free. kn For the( most− ) part it is easier to work with the Stiefel coordinates¨ on the Grassman- n 2 nian, rather than the k Plucker¨ coordinates¨ that we won’t bother introducing. n As we explain below, much the same construction gives an atlas on Fl n1; n2; : : : ; nd; C , ′ restricting the surjective rowspan map to slices Uλ that together cover the target. The difference is that the group we’re dividing by, instead of GLk C , is the( group P of block) lower triangular matrices in GLnd C with diagonal blocks of size n1; n2 n1; : : : ; nd nd−1. ( n) We will need a finer analogue of the covering Stiefel k; C [n] Uλ. Let M λ∈ k n ( ) − − [n] Stiefel nd; C be a matrix we’re making sure not to exclude. Start by picking λd ( ) = ⋃ nd ∈ such that M’s submatrix with columns λd (and all nd rows) is invertible, exactly as we did ( ) λd ∈ before. But now we also pick a subset λd 1 such that M’s submatrix with columns − nd−1 λd−1 λd−1 (and first nd−1 rows, a top submatrix) is invertible, then similarly λd−2 n , etc. ∈ d−2 Encode this sequence λ1 λ2 ::: λd n of subsets as a string λ, whose ith letter is ∈ min j j λi (considering λd+1 n ). To such a string we associate the manifolds ( ⊆n ⊆ ⊆ ⊆ [ ]) Uλ N Stiefel nd; det N’s top submatrix with columns λi 0; i 1 : : : d { ∶ ∉ } C = [ ] N’s top submatrix with columns λi ′ ∶= { ∈ ( n) ∶ ( ) ≠ ∀ = } Uλ N Stiefel nd; C is a permutation matrix, i 1 : : : d ⎧ 1 1 n ; n ⎫ ⎪ whose s run NW/SE in rows i−1 i ⎪ As in∶ the= ⎨ Grassmannian∈ ( case,) ∶ ∀ = ⎬ ⎪ ⎪ ⎩ n [ + ] ⎭ these Uλ cover Stiefel nd; C (the construction of λ above made sure to hit M), they’re invariant under the left action of P (the block lower triangular group de- ● fined( above),) ( ) ′ ● the submanifold Uλ serves as a system of P-orbit representatives in Uλ, so n rowspan ′ U′ Fl n ; n ; : : : ; n ; the maps Uλ λ 1 2 d C form an atlas, again easily ● checked to be algebraic. ● S ∶ → ( ) n 1.2. The Bruhat decomposition of Gr k; C . The left GLk C action on Mk×n is a fa- miliar topic in first linear algebra classes, under the name “row reduction” or “Gaussian elimination”. The principal result there( concerning) it is the construction( ) of a discontinuous system of orbit representatives, the reduced row-echelon forms (or RREF) such as 0 0 1 0 0 0 0 0 0 0 0 0 1 0 0 0 ⎡ ⎤ ⎢0 0 0∗ 0 ∗0 0 1 ∗ 0 0 ∗⎥ ⎢ ⎥ ⎢0 0 0 0 0 0 0∗ 0 1 0 ∗⎥ ⎢ ⎥ ⎢ ⎥ ⎢0 0 0 0 0 0 0∗ 0 0 1 ∗⎥ ⎢ ⎥ ⎢00000000000∗⎥ ⎢ ⎥ ⎢00000000000∗⎥ ⎢ ⎥ ⎢ ⎥ ⎢ ⎥ characterized by the properties⎢ ⎥ The zero rows are at⎣ the bottom ⎦ The nonzero rows start with a 1, called a pivot ● The pivotal 1s run NW/SE ● 2They are coordinates¨ on the smallest GL V -equivariant embedding of Gr k; V into projective space, ● k k via the map V1 Alt V1 P Alt V . In particular, they let one study the Grassmannian as a subvariety of a simpler space, rather than as a quotient. ( ) ( ) ↦ ∈ ( ) SCHUBERT CALCULUS AND QUIVER VARIETIES 5 Above each pivotal 1 (and below, automatically) is all 0s.
Details
-
File Typepdf
-
Upload Time-
-
Content LanguagesEnglish
-
Upload UserAnonymous/Not logged-in
-
File Pages42 Page
-
File Size-