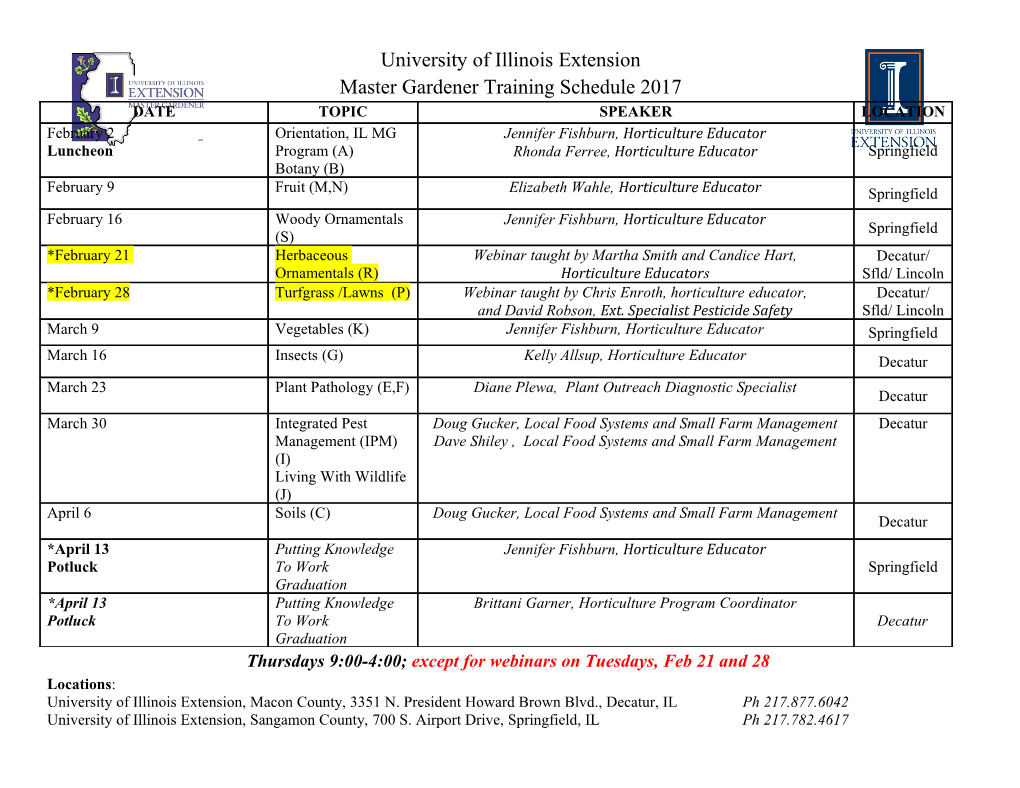
Glycogen metabolism Subtopics Introduction Degradation of glycogen: glycogen phosphorylase, glycogen debranching enzyme, phosphoglucomutase and glucose-6 phosphatase Glycogen synthesis: UDP-glucose pyrophosphorylase, glycogenin, glycogen synthase, glycogen branching enzyme and significance of glycogen branching Thermodynamics of glycogen metabolism Control of glycogen metabolism: allosteric regulation and bicyclic cascade of glycogen phosphorylase and glycogen synthase Integration of glycogen metabolism: cAMP-dependent phosphorylation cascade (G-proteins, adenylate cyclase, protein kinase A, phosphorylase kinase) and calcium-dependent phosphorylation cascade (Calmodulin and phosphorylase kinase) Signal amplification and signal termination by phosphoprotein phosphatase 1 Insulin and the well-fed state, maintenance of blood glucose level and glucose transporters (GLUT1-GLUT5) Glucagon and the fasting state Epinephrine and the stress response, the fructose-2,6-bisphosphate control system Glycogen storage diseases: Deficiencies of G6Pase, glucosidase, debranching enzyme, branching enzyme and muscle phosphorylase. 1 | P a g e Glycogen metabolism Introduction Glucose cannot be stored because high concentrations of glucose disrupt the osmotic balance of the cell. Higher organisms protect themselves from potential fuel shortage and osmotic damage by polymerizing excess glucose into glycogen. Glycogen is a high molecular weight storage polymer of glucose that can be readily mobilized in times of metabolic demand. It is predominantly α (1→4)-linked glucose polymer with α (1→6) branches every 8 to 14 residues. Glycogen is stored as large cytoplasmic particles in the form of hydrated granules. These cytoplasmic granular particles contain the enzymes that metabolize glycogen, as well as the machinery for regulating these enzymes. Glycogen forms a left- handed helix with 6.5 glucose residues per turn, similar to α-amylose. No matter how big, glycogen has but one reducing end. Glucose units from glycogen are mobilized by their sequential removal from their nonreducing ends. Storing energy as glucose polymers is common to all forms of life. It is present in eukaryotes, bacteria and archae. In plants, the glucose storage substance is starch. Glucose released from glycogen, unlike triacylglycerols from lipids, can be metabolized in the absence of oxygen and thus it can supply energy for anaerobic activity. Glycogen is a preferred source of energy for sudden, strenuous activity of muscles which cannot mobilize fats as rapidly as glycogen. The fatty acid residues of lipids cannot also be catabolized anaerobically. Furthermore, animals cannot convert fatty acids to glucose so fat metabolism alone cannot adequately maintain essential blood glucose levels. The two major storage tissues for glycogen storage in animals are the liver and skeletal muscles. Liver glycogen serves as a reservoir of glucose for other tissues when dietary glucose is not available. It maintains glucose homeostasis of the organism as a whole. In mammals, the neurons of brain have virtually no stored source of energy and they cannot use fatty acids as fuel. They are rather completely dependent on a constant supply of glucose from the blood. The circulating blood keeps the brain supplied with glucose, which is virtually the only fuel used by the brain, except during prolonged starvation. Hence glycogen in the liver is especially important to maintain the blood-glucose levels as required to meet the needs of the organism as a whole. In contrast, glycogen in muscle is utilized to meet the energy needs of only the selfish muscle. Muscle glycogen serves as a reservoir for muscle cells to provide a quick source of energy for either aerobic or anaerobic metabolism. Muscle contraction is driven by generating ATP through glycolysis of glucose. Muscle glycogen can be exhausted in less than 2 | P a g e an hour during vigorous activity. The enzymes in liver and muscle differ in subtle yet important ways that reflect the different roles of glycogen in the two tissues. Glycogen breakdown Glycogenolysis or glycogen breakdown is a catabolic pathway from glycogen to glucose-6-phosphate. It provides the energy essential to oppose the forces of entropy and biosynthetic precursors. Glycogen degradation comprises three steps catalyzed by three enzymes: the release of glucose-1-phosphate from glycogen by glycogen phosphorylase, glycogen remodeling by glycogen debranching enzyme and the conversion of glucose-1-phosphate (G1P) into glucose-6-phosphate (G6P) by phosphoglucomutase. Glycogen phosphorylase (or phosphorylase) catalyzes the sequential removal of glucosyl residues from the nonreducing ends of the glycogen substrate by the addition of orthophosphate (Pi) to yield G1P. It hydrolyzes α (1→4)-linked glucosyl units. The cleavage of a bond through the addition of a phosphate group is referred as phosphorolysis or phosphorolytic cleavage. Phosphorylase can only release a glucose unit that is at least five units from a branch point. Two activities are required to remodel glycogen by converting the branched structure into a linear form and permit further degradation by glycogen phosphorylase. These are a transferase and α-1,6-glucosidase activities. Glycogen debranching enzyme removes glycogen’s branches to allow the phosphorylase reaction to go to completion. It catalyzes both the transfer of three-residue chains onto the nonreducing ends of other chains and the hydrolysis of the remaining α(1→6)-linked glucosyl unit to yield glucose. The cleavage of a bond by addition of water is called hydrolysis or hydrolytic cleavage. Phosphorolysis of glycosidic bonds differs from hydrolysis by amylase during intestinal degradation of dietary glycogen and starch. In phosphorolysis, some of the energy of the glycosidic bond is preserved in the formation of the phosphate ester in G1P. G1P released from glycogen breakdown can be readily converted into G6P by phosphoglucomutase. G6P is suitable form for further metabolism. A. Glycogen phosphorylase Glycogen phosphorylase catalyzes the cleavage of an α(1→4) glycosidic bond between two glucose residues at any nonreducing end of glycogen. The reaction removes the terminal glucose residue as α-D- G1P through attack by inorganic phosphate (Pi). It catalyzes the controlling step in glycogen breakdown. Phosphorylase is a dimer of two identical subunits localized in the cytosol. Each subunit is compactly folded into an amino-terminal domain with a glycogen-binding site and a carboxyl-terminal domain. Phosphorylase is regulated both by both allosteric interactions and post-translational modification. Each 3 | P a g e subunits of the phosphorylase dimer can interconvert between a less active tensed (T) state and a more active relaxed (R) state. The T state is less active because the catalytic site is partly blocked by a loop. Allosteric inhibitors of phosphorylase such as ATP, G6P, and glucose favor the formation of the T state whereas allosteric activator such AMP favors the formation of the R-state. Covalent modification by phosphorylation on Ser-14 of each subunit favors the structure of the R state. Phosphorylase exists in two types of interchangeable conformers: phosphorylase b (catalytically inactive) and phosphorylase a (catalytically active). Both forms undergo equilibrium between the T and R states in which the unmodified form exist predominantly in the T state whereas the phosphorylated form exists predominantly in the R state. Allosteric regulators interact differently with the phospho- and dephosphoenzymes. Moreover, there are two isozymic forms of glycogen phosphorylase encoded by different genes: one specific to liver and one specific to skeletal muscle. In human beings, the liver and muscle forms are approximately 90% identical in amino acid sequence, yet the 10% difference results in subtle but important shifts in the stability of different forms of the enzyme. The a-form in liver can be reverted to the low-activity T state upon binding of glucose to its active site. The a-form in liver functions as glucose sensor. On the other hand, the b form in muscle can be activated by the binding of AMP, an effect counteracted by ATP and glucose 6-phosphate. Glycogen phosphorylase provides a clear example of the use of enzymatic isoforms to establish tissue-specific regulatory properties. The special challenge in the glycogen phosphorylase reaction is the exclusion of water from the active site. To this end, phosphorylase contains pyridoxal-5’-phosphate (PLP) group which functions as an essential cofactor. PLP is a derivative of pyridoxine (vitamin B 6). The aldehyde group of PLP forms a Schiff-base linkage with a Lys 680 of the enzyme. It is similarly linked to a variety of enzymes involved in transamination reactions. A Schiff base, also called an imine, is formed by the reaction of a primary amine with an aldehyde or ketone to produce a carbon–nitrogen double bond. The glycogen-binding site of phosphorylase is 30 Å away from the catalytic site on the surface which is connected to the catalytic site by a narrow crevice that can accommodate four or five sugar residues in a chain. However, this crevice is too narrow to admit branched oligosaccharides. The large separation between the substrate binding site and the catalytic site allows the enzyme to cleave many residues before the enzyme must bind the glycogen substrate again. An enzyme that can catalyze many reactions without having to dissociate and reassociate after each catalytic cycle is said to
Details
-
File Typepdf
-
Upload Time-
-
Content LanguagesEnglish
-
Upload UserAnonymous/Not logged-in
-
File Pages32 Page
-
File Size-