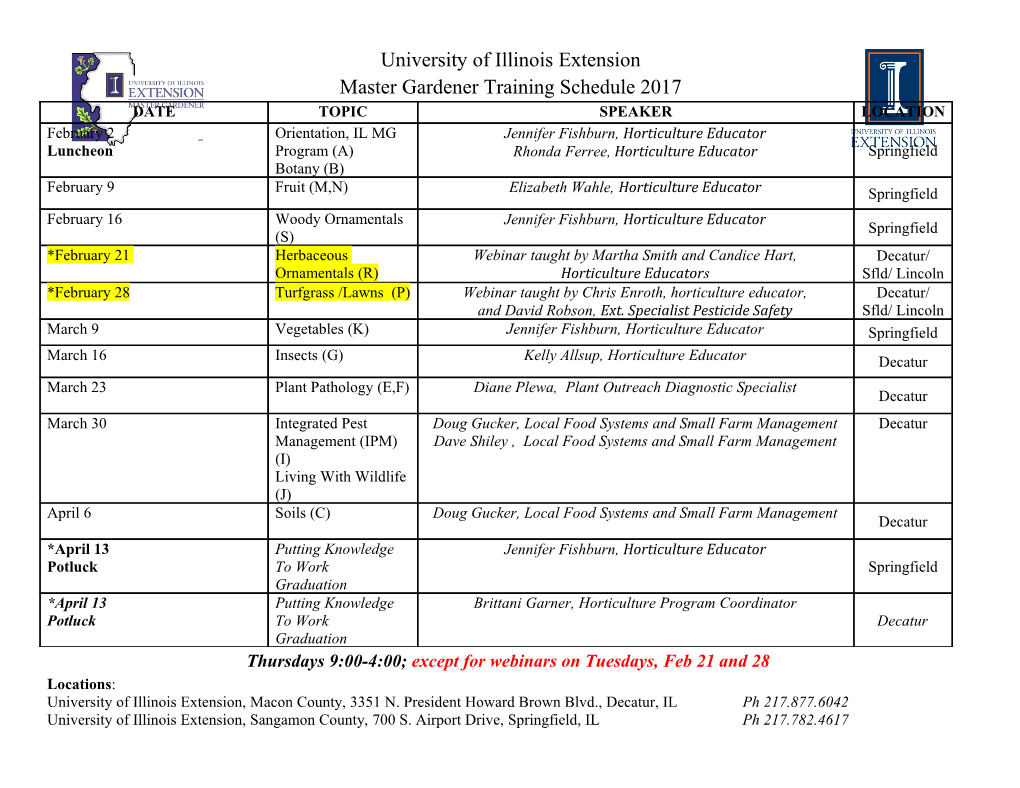
10928 Langmuir 2004, 20, 10928-10934 Halogen-Substituted Thiophenol Molecules on Cu(111) K. L. Wong,† X. Lin,† K.-Y. Kwon,† G. Pawin,† B. V. Rao,† A. Liu,† L. Bartels,*,† S. Stolbov,‡ and T. S. Rahman*,‡ Pierce Hall, University of California, Riverside, California 92521, and Department of Physics, Kansas State University, Manhattan, Kansas 66506 Received July 16, 2004. In Final Form: September 19, 2004 Para-halosubstituted thiophenols (X-TPs, where X is Br, Cl, or F) form ordered islands and monolayers on Cu(111) at temperatures as low as 81 K. At incomplete coverages, all X-TPs adsorb with the dehydrogenated thiol group attached to the substrate and the substituted ring inclined toward the surface, as verified experimentally and theoretically. The structure of ordered islands has a pronounced dependence on the nature of the halogen substituent: while unsubstituted TP and pentafluoro-TP molecules do not self-assemble into extended ordered patterns at 81 K, X-TP molecules form a range of different structures which depend both on the size and electronegativity of the substituent, as well as on the coverage. Introduction TP film forms a commensurate (x13 ×x13)R30° structure on Au(111).32 However, Dhirani et al. report that TP does The study of self-assembled monolayers (SAMs) is 15 motivated by a large array of potential applications that not form an ordered SAM in their STM study. In addition, - includes molecular electronic devices,1,2,3 chemical sen- a variety of results concerning the sulfur substrate sors,4 corrosion inhibitors,5 biocompatible coatings,6 etc. bonding, the precise orientation of the phenyl ring with respect to the surface, and the two-dimensional film While the majority of the studies on thiol-based SAMs 16-19 address dense coverages7,8 and the mechanism of SAM structure can be found in the literature. Biphenylic formation,9 a limited number of studies has investigated SAMs were found to lie close to the surface plane at lower coverage and to tilt toward the surface normal at higher the evolution of film structure with increasing coverage 20 and the interplay between intermolecular and molecule- coverage. substrate interactions.10 Poirier and Pylant11 proposed In ultrahigh vacuum (UHV), a clean Au(111) surface that SAMs form in a two-step mechanism driven by strong forms a herringbone reconstruction, which leads to dis- molecule-substrate interaction while being governed by similar surface sites and provides a complication for the a dynamical rather than an energetic equilibrium. investigation of the SAM formation process. Cu(111) The most popular SAM system is organothiols on shares many physical and chemical properties with 21 Au(111) due to their facile preparation and good stability Au(111) as far as thiol interactions are concerned, yet even in air.12 Experiments show a sequence of different it offers the advantages of the absence of a reconstruction. adsorbate patterns13,14 depending on coverage. Compared At the same time, it is structurally stable upon thiol to the alkanethiols, arenthiols have been studied less intensely. In the case of the simplest arenethiol, thiophenol (15) Dhirani, A. A.; Zehner, R. W.; Hsung, R. P.; Guyot-Sionnest, P.; Sita, L. R. J. Am. Chem. Soc. 1996, 118, 3319. (TP), Wan et al. concluded that the phenyl ring is tilted (16) Szafranski, C. A.; Tannaer, W.; Laibinis, P. E.; Garrell, R. about 30° from the surface normal and that a well-ordered Langmuir 1998, 14, 3570. (17) Carron, K. T.; Hurley, G. J. Phys. Chem. 1991, 95, 9979. * Authors to whom correspondence should be addressed. E- (18) Hasan, M.; Bethell, D.; Brust, M. J. Am. Chem. Soc. 2002, 124, 1132. mail: [email protected] (L.B.); [email protected] (19) Nuzzo, R. G.; Zegarski, B. R.; Dubois, L. H. J. Am. Chem. Soc. (T.S.R.). 1987, 109, 733. † University of California. (20) Leung, T. Y. B.; Schwartz, P.; Scoles, G.; Schreiber, F.; Ulman, ‡ Kansas State University. A. Surf. Sci. 2000, 458, 34. (1) Reed, M. A.; Zhou, C.; Muller, C. J.; Burgin, T. P.; Tour, J. M. (21) Ulman, A. Chem. Rev. 1996, 96, 1533. Science 1997, 278, 252. (22) Kariapper, M. S.; Grom, G. F.; Jackson, G. J.; McConville, C. F.; (2) Mantooth, B. A.; Weiss, P. S. Proc. IEEE 2003, 91, 1785. Woodruff, D. P. J. Phys.: Condens. Matter 1998, 10, 8661. (3) Collier, C. P.; Jeppesen, J. O.; Luo, Y.; Perkins, J.; Wong, E. W.; (23) Toomes, R. L.; Polcik, M.; Kittel, M.; Hoeft, J. T.; Sayago, D. I.; Heath, J. R.; Stoddart, J. F. J. Am. Chem. Soc. 2001, 123, 12632. Pascal, M.; Lamont, C. L. A.; Robinson, J.; Woodruff, D. P. Surf. Sci. (4) Flink, S.; van Veggel, F.; Reinhoudt, D. N. Adv. Mater. 2000, 12, 2002, 513, 437. 1325. (24) Jackson, G. J.; Woodruff, D. P.; Jones, R. G.; Singh, N. K.; Chan, (5) Scherer, J.; Vogt, M. R.; Magnussen, O. M.; Behm, R. J. Langmuir A. S. Y.; Cowie, B. C. C.; Formoso, V. Phys. Rev. Lett. 2000, 84, 119. 1997, 13, 7045. (25) Rieley, H.; Kendall, G. K.; Chan, A.; Jones, R. G.; Ludecke, J.; (6) Prime, K. L.; Whitesides, G. M. J. Am. Chem. Soc. 1993, 115, Woodruff, D. P.; Cowie, B. C. C. Surf. Sci. 1997, 392, 143. 10714. (26) Nara, J.; Kino, J.; Kobayashi, N.; Tsukada, M.; Ohno, T. Thin (7) Poirier, G. E. Chem. Rev. 1997, 97, 1117. Solid Films 2003, 438, 221. (8) Schreiber, F. Prog. Surf. Sci. 2000, 65, 151. (27) Vondrak, T.; Cramer, C. J.; Zhu, X. Y. J. Phys. Chem. B 1999, (9) Schwartz, D. K. Annu. Rev. Phys. Chem. 2001, 52, 107. 103, 8915. (10) Barrena, E.; Palacious-Lidon, E.; Munuera, C.; Torrelles, X.; (28) Madhavan, K.; Muralidharan, S.; Iyer, S. V. Anti-Corros. Methods Ferrer, S.; Jonas, U.; Salmeron, M.; Ocal, C. J. Am. Chem. Soc. 2004, Mater. 1998, 45, 227. 126, 385. (29) Frey, S.; Stadler, V.; Heister, K.; Eck, W.; Zharnikov, M.; Grunze, (11) Poirier, G. E.; Pylant, E. E. Science 1996, 272, 1145. M.; Zeysing, B.; Terfort, A. Langmuir 2001, 17, 2408. (12) Poirier, G.; Tarlov, M. M. Langmuir 1994, 10, 2853. (30) Whelan, C. M.; Barnes, C. J.; Gregoire, C.; Pireaux, J. J. Surf. (13) Azzam, W.; Cyganik, P.; Witte, G.; Buck, M.; Wo¨ll, C. Langmuir Sci. 2000, 454, 67. 2003, 19, 8262. (31) Sawaguchi, T.; Mizutani, F.; Yoshimoto, S.; Taniguchi, I. (14) Schreiber, F.; Eberhardt, A.; Leung, T. Y. B.; Schwartz, P.; Electrochim. Acta 2000, 45, 2861. Wetterer, S. M.; Larvic, D. J.; Berman, L.; Fenter, P.; Eisenberger, P.; (32) Wan, L. J.; Terashima, M.; Noda, H.; Osawa, M. J. Phys. Chem. Scoles, G. Phys. Rev. B 1998, 57, 12476. B 2000, 104, 3563. 10.1021/la048208b CCC: $27.50 © 2004 American Chemical Society Published on Web 11/05/2004 Halogen-Substituted Thiophenol Molecules on Cu(111) Langmuir, Vol. 20, No. 25, 2004 10929 in its own UHV chambers. All measurements described here proceeded at 80-85 K, as measured by silicon diodes attached to the STMs (with exception of the data of Figure 4, which was obtained at 15 K). The base pressure of the STM chambers is consistently below 6 × 10-11 Torr (measured with a nitrogen- calibrated hot-cathode ion gauge). The STM tips were made by electrochemical etching of a tungsten wire followed by hot (white glow) annealing in vacuo. The Cu(111) samples (Monocrystals and MaTecK GmbH) are prepared using cycles of standard argon ion sputtering (up to 3 kV) and annealing (600 K). Sample cleanliness is ascertained by STM both at room temperature and after cooling to cryogenic temperatures. The samples were considered clean if no more Figure 1. 1. Ball and stick models of the molecules of this than 1/10 000 of a monolayer of contamination was found on the study (clockwise from the top left corner): Thiophenol (C6SH6), surface (corresponding to one or two contaminants per image p-fluorothiophenol (C6FSH5), p-chlorothiophenol (C6ClSH5), frame). p-bromothiophenol (C6BrSH5), and pentafluorothiophenol (C6F5- Deposition of the thiophenols onto the sample for STM purpose SH). All STM images represent molecules, whose thiol (-SH) proceeded by backfilling of the turbo-pumped chamber to 1 × group is dehydrogenated, so that the S atom is not bound to a 10-9 Torr using a leak valve while the sample was protected hydrogen atom but rather to the substrate. behind the cryostat thermal shields. A mass spectrum was taken to confirm the purity of the reactant. Subsequently, all filaments adsorption22 at low temperatures. Alkanethiols adsorb on of the chamber were turned off, the sample was extracted from higher coordinated sites23-25 on Cu(111). Due to its the cryostat, and it was exposed to the backfilled chamber for up properties, Cu(111) is a well-suited model system for the to 30 s at a time. The short exposure time ensures constant cryogenic temperature of the sample, as ascertained by virtually investigation of the evolution of SAM films and their drift-free imaging immediately after sample exposure. Repeated structural properties with increasing coverage. exposure cycles were used to reach the coverages indicated here, This study focuses on the adsorption of thiophenols as necessary. (benzenthiols) (TP) which are either unsubstituted or carry Where mentioned, annealing of the adsorbate layer involved a halogen (F, Cl, Br) in the para position or in all positions removal of the sample from the cryostat for a prolonged period of the ring (Figure 1). TPs are one of the simplest model (>1 h), which allows it to warm gradually to room temperature. systems for future molectronic building blocks,26 as they During this period, all filaments in the chamber were switched contain the common thiol substrate linker and an aromatic off to avoid sample contamination.
Details
-
File Typepdf
-
Upload Time-
-
Content LanguagesEnglish
-
Upload UserAnonymous/Not logged-in
-
File Pages7 Page
-
File Size-