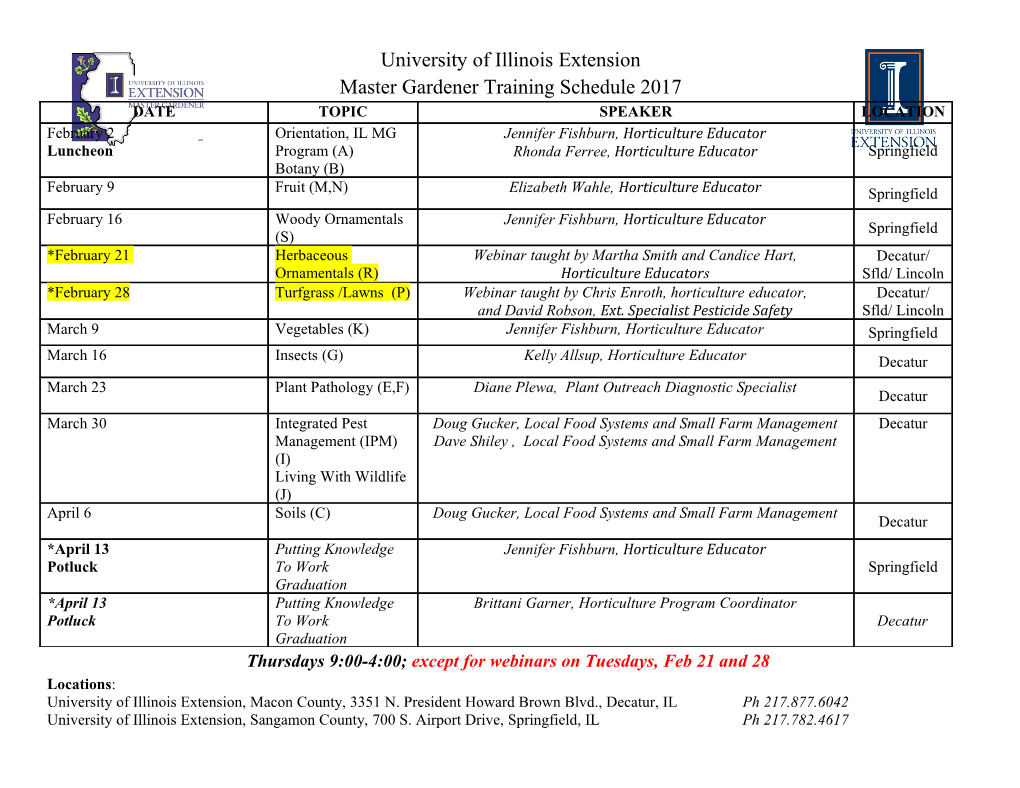
Structure of a quinohemoprotein amine dehydrogenase with an uncommon redox cofactor and highly unusual crosslinking Saumen Datta*, Youichi Mori†, Kazuyoshi Takagi‡§, Katsunori Kawaguchi‡, Zhi-Wei Chen*, Toshihide Okajima†, Shun’ichi Kuroda†, Tokuji Ikeda‡, Kenji Kano‡, Katsuyuki Tanizawa†¶, and F. Scott Mathews*¶ʈ *Department of Biochemistry and Molecular Biophysics, Washington University School of Medicine, St. Louis, MO 63110; †Department of Structural Molecular Biology, Institute of Scientific and Industrial Research, Osaka University, Ibaraki, Osaka 567-0047, Japan; and ‡Division of Applied Life Sciences, Graduate School of Agriculture, Kyoto University, Sakyo-ku, Kyoto 606-8502, Japan Edited by Perry A. Frey, University of Wisconsin, Madison, WI, and approved October 1, 2001 (received for review August 14, 2001) The crystal structure of the heterotrimeric quinohemoprotein The Gram-negative chemoorganotrophic bacterium Paracoc- amine dehydrogenase from Paracoccus denitrificans has been de- cus denitrificans contains two amine dehydrogenases in its termined at 2.05-Å resolution. Within an 82-residue subunit is periplasm, one being the well-known TTQ-containing MADH contained an unusual redox cofactor, cysteine tryptophylquinone and the other a quinohemoprotein amine dehydrogenase (CTQ), consisting of an orthoquinone-modified tryptophan side (QHNDH) that is preferentially induced by growth on n- chain covalently linked to a nearby cysteine side chain. The subunit butylamine (10). QHNDH was reported to be a heterodimer of is surrounded on three sides by a 489-residue, four-domain subunit 96-kDa molecular mass. The large subunit of 60 kDa appeared that includes a diheme cytochrome c. Both subunits sit on the to contain one heme c per molecule and to stain positively for surface of a third subunit, a 337-residue seven-bladed -propeller quinone-dependent redox cycling. The smaller subunit of 36 kDa that forms part of the enzyme active site. The small catalytic showed no redox activity. Partial reduction of the enzyme by subunit is internally crosslinked by three highly unusual covalent dithionite gave a semiquinone radical EPR signal similar to that cysteine to aspartic or glutamic acid thioether linkages in addition of TTQ in MADH. Cytochrome c550 has been shown to be the to the cofactor crossbridge. The catalytic function of the enzyme as natural electron acceptor for QHNDH (11). A similar QHNDH well as the biosynthesis of the unusual catalytic subunit is has been isolated from Pseudomonas putida, but apparently with discussed. somewhat different subunit composition and with exogenous electron acceptor specificity for azurin (12). nzymes that catalyze the oxidation of biological amines use In this paper we describe the crystal structure and gene Ea variety of redox cofactors to temporarily store the reducing sequence of QHNDH from P. denitrificans, revealing that it equivalents derived from the substrate before passing them on to comprises three nonidentical subunits, with the largest contain- exogenous one- or two-electron acceptors such as a cytochrome, ing two heme c groups, and the smallest containing the unusual a cupredoxin, or molecular oxygen. The majority of such en- quinone cofactor cysteine tryptophylquinone (CTQ). More sur- zymes employ a flavin (FMN or FAD) as prosthetic group, but prisingly, the small subunit contains three additional chemical some, such as copper amine oxidase (CAO) and methylamine crosslinks involving a cysteine side chain bridged by a thioether dehydrogenase (MADH) contain a quinone cofactor (1). In the bond to a carboxylic acid side chain, either aspartate or case of CAO, the cofactor is topaquinone (TPQ) (2) or lysine glutamate. tyrosylquinone (LTQ) (3), whereas for MADH, the cofactor is Materials and Methods tryptophan tryptophylquinone (TTQ) (4) (Fig. 1). All three quinone cofactors are unusual because each of them is derived Crystal Structure Analysis. QHNDH was isolated and purified as from one or two amino acid side chains that are encoded by the described previously (10). Microcrystals were obtained by sit- Ϸ ⅐ Ϫ1 genome and are chemically modified posttranslationally (5). ting-drop vapor diffusion at 293 K. The protein ( 15 mg ml in ⅐ Another class of quinoenzyme, as represented by methanol 10 mM Tris HCl buffer, pH 7.5) was mixed with an equal volume ͞ dehydrogenase, is involved in the oxidation of alcohols and of reservoir solution (100 mM sodium citrate buffer, pH 5.6 ͞ sugars, and contains pyrroloquinoline quinone (PQQ) as redox 18% PEG 4,000 21% t-butyl alcohol) and allowed to equilibrate. cofactor (6). In these enzymes, the PQQ is bound noncovalently and is incorporated from an intracellular or extracellular pool This paper was submitted directly (Track II) to the PNAS office. that is maintained by a separate biosynthetic pathway. Abbreviations: CAO, copper amine oxidase; CTQ, cysteine tryptophylquinone; MADH, The CAO enzymes are found in both eukaryotes and pro- methanol dehydrogenase; PQQ, pyrroloquinoline quinone; QHNDH, quinohemoprotein karyotes and use molecular oxygen as the exogenous electron amine dehydrogenase; SAM, S-adenosylmethionine; TPQ, topaquinone; TTQ, tryptophan acceptor (7). The remaining quinoenzymes, containing TTQ or tryptophylquinone; Trq, tryptophylquinone; ESI, electrospray ionization; NPH, PQQ, are bacterial in origin and are periplasmic. Neither of them nitrophenylhydrazine. directly reduces molecular oxygen, but rather they feed the Data deposition: The gene sequence and the atomic coordinates of QHNDH have been deposited in GenBank (accession no. AB063330) and the Protein Data Bank, www.rcsb.org substrate-derived electrons one at a time into the terminal (PBD ID code 1JJU), respectively. oxidase electron transport system of the periplasm, either di- §Present address: Department of Applied Chemistry, Faculty of Science and Engineering, rectly or through the mediation of cupredoxin or cytochrome Ritsumeikan University, Kusatsu, Shiga 525-8577, Japan. electron-carrier proteins (1, 8). Some PQQ enzymes are quino- ¶K. Tanizawa and F. S. Matthews are both senior authors of this paper. hemoproteins, because they contain both PQQ and one or more ʈTo whom reprint requests should be addressed. E-mail: [email protected]. heme moieties located in the same or different subunits, and The publication costs of this article were defrayed in part by page charge payment. This undergo intramolecular electron transfer before being reoxi- article must therefore be hereby marked “advertisement” in accordance with 18 U.S.C. dized by an exogenous electron acceptor (9). §1734 solely to indicate this fact. 14268–14273 ͉ PNAS ͉ December 4, 2001 ͉ vol. 98 ͉ no. 25 www.pnas.org͞cgi͞doi͞10.1073͞pnas.241429098 Downloaded by guest on September 27, 2021 0.1 mM DTT. A protein band of about 9 kDa, detected by quinone-dependent redox cycling (24) after electroblotting onto a poly(vinylidene difluoride) membrane, was eluted from the finely ground gel pieces in 20 mM ammonium bicarbonate containing 8 M urea, dialyzed thoroughly against distilled water, and analyzed for the content of free SH groups with 0.2 mM 5,5Ј-dithiobis(2-nitorobenzoic acid) by monitoring the increase in absorbance at 412 nm. For reductive carboxymethylation, the ␥ subunit polypeptide was first incubated for4hwith0.5mM DTT in 50 mM Tris buffer (pH 8.0) containing 6 M guanidine hydrochloride under N2 gas stream, then reacted for1hwitha freshly prepared solution of iodoacetic acid (1 mM). Amino acid composition of the peptide was determined with an amino acid analyzer after hydrolysis at 110°C for 24 h in 6 M HCl containing 0.2% phenol. For isolation of the quinone-containing peptide, the enzyme was modified with excess p-nitrophenylhydrazine (NPH) to protect the quinone group, and digested with trypsin for 24 h and then with Pronase for4hin0.15 M NH4HCO3 (pH Fig. 1. Chemical structures of the quinone cofactors PQQ, TPQ, lysine 8.0) and 2.5 M urea. The peptide containing the NPH-quinone tyrosylquinone (LTQ), and TTQ. The structure of the newly identified cofactor, hydrazone was isolated by reverse-phase HPLC by monitoring cysteine tryptophylquinone (CTQ), is also shown. the 464-nm absorbance derived from the hydrazone. Sequence analysis of the peptide with or without prior reductive carboxy- Large crystals (up to 0.5 ϫ 0.5 ϫ 0.4 mm3) were obtained by methylation was done with an Applied Biosystems 476A protein macroseeding. sequencer. Electrospray ionization (ESI)-MS͞MS was carried X-ray data were collected at Ϸ100 K from flash frozen out on a hybrid quadrupole orthogonal acceleration tandem crystals, cryo-protected with mineral oil, on an R-axis IV image mass spectrometer (Q-TOF; Micromass, Manchester, U.K.). plate system mounted on a Rikagu RU-200 rotating anode x-ray MS͞MS data were processed by the maximum entropy data generator operating at 50 kV and 100 mA. Data were processed enhancement program MAXENT 3 (Micromass). The resultant with the HKL package (13). Two forms of native crystals (native I and native II) were identified, each with one molecule in the asymmetric unit, but with slightly different cell dimensions. Table 1. Data collection, phasing, and refinement statistics Crystals soaked with ethylmercury phosphate, platinum ethyl- Native I Native II EMP PEDD MMC enediamine dichloride, and methylmercury chloride produced interpretable diffraction changes. Two Fe positions were located Resolution, Å 30–2.04 30–2.6 30–3.0 30–3.0 30–3.0 from the anomalous difference Patterson map from a highly Unit cell redundant native data set (native II). Initial phase information a, Å 99.49 99.49 99.11 98.80 98.56 for reflections up to 2.6-Å resolution was obtained by multiple c, Å 214.49 210.38 211.58 209.16 213.07 isomorphous replacement (MIRAS) using the anomalous con- No. unique reflections 58,964 31,819 19,897 19,960 18,235 tribution of the native data set (native II) and the isomorphous Rsym* 6.2 5.1 7.6 9.4 10.2 plus anomalous contributions from the three derivatives. The (Outer shell) (21.7) (9.4) (11.7) (12.8) (22.6) Completeness, % 86.4 96.4 91.4 92.3 84.5 program SOLVE (14) was used for all of the MIRAS calculation.
Details
-
File Typepdf
-
Upload Time-
-
Content LanguagesEnglish
-
Upload UserAnonymous/Not logged-in
-
File Pages6 Page
-
File Size-