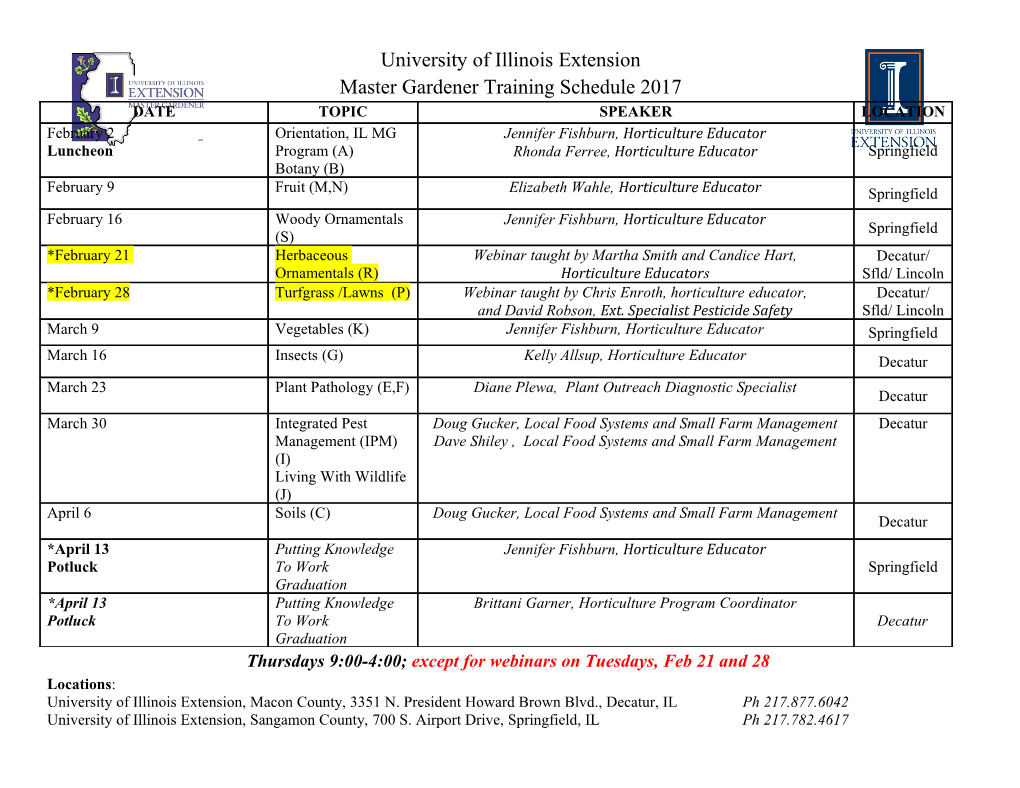
Use of small animal PET/MRI for internal radiation dose assessment Dissertation zur Erlangung des akademischen Grades Dr. rer. med. an der Medizinischen Fakult¨at der Universit¨at Leipzig eingereicht von: M.Sc. Mathias Kranz Geburtsdatum/Geburtsort: 21.08.1986/Bad Salzungen angefertigt am/in: Universit¨atsklinikum Leipzig (UKL), Klinik und Poliklinik fur¨ Nuklearmedizin sowie Helmholtz-Zentrum Dresden-Rossendorf (HZDR), Forschungsstelle Leipzig, Abteilung Neuroradiopharmaka Betreuer: UKL: Prof. Dr. -Ing. Bernhard Sattler HZDR: Prof. Dr. Peter Brust Beschluss uber¨ die Verleihung des Doktorgrades vom: Table of Content 1 Introduction ................................................... 1 1.1 Radiation dosimetry . 2 1.1.1 Quantities and units . 3 1.1.2 Dose calculation . 5 1.1.3 Dose limits . 6 1.2 Preclinical dosimetry . 7 1.2.1 Small animal imaging . 8 1.2.2 Small animal PET/MRI for image based dosimetry . 9 1.2.3 Correction methods of preclinical data used for human dose assessment.......................................... 11 1.3 Objective of the study . 12 2 Publications ................................................... 15 2.1 Study 1: Internal Dose Assessment of (-)-[18F]flubatine, Comparing Animal Model Datasets of Mice and Piglets with First-in-Human Results .............................................. 15 2.2 Study 2: Radiation Dosimetry of the α4β2 Nicotinic Receptor Ligand (+)-[18F]flubatine, Comparing Preclinical PET/MRI and PET/CT to First-in-Human PET/CT Results . 24 2.3 Study 3: Evaluation of the Enantiomer Specific Biokinetics and Radiation Doses of [18F]fluspidine - A New Tracer in Clinical Translation for Imaging of σ1 Receptors ......................... 42 3 Summary of the dissertation .................................... 57 3.1 Summary and conclusions . 57 References ................................................... ...... 63 4 Appendix ................................................... ... 71 4.1 Erkl¨arungen uber¨ den wissenschaftlichen Beitrag zu den Publikationen 71 4.2 Erkl¨arung uber¨ die eigenst¨andige Abfassung der Arbeit . 75 4.3 Lebenslauf .......................................... ......... 76 4.4 Publikationsverzeichnis . 79 4.5 Danksagung.......................................... 87 1 Introduction The health risk of biological specimens exposed to ionizing radiation became appa- rent shortly after the discovery of ionizing radiation (X-rays) in 1895 by Wilhelm Conrad Roentgen [1, 2, 3, 4, 5]. High radiation doses1 which exceed a certain thres- hold level damage living cells which finally results in cell death (deterministic ef- fects). However, cell information might be modified even by lower doses (stochastic effects2). This modification is usually repaired by intrinsic cell mechanisms such as DNA repair or the elimination of damaged cells [8]. If this process is incorrect, the modification (mutation) will be transmitted to other cells and might cause cancer. Notably, the world population is constantly exposed to natural- (terrestrial, solar etc.) and man-made ionizing radiation of different kind and sources. The latter includes nuclear bomb tests, nuclear power plants (and accidents) and the use of radiation for clinical applications. The medical use and application of ionizing radiation (i.e. α-, β- or γ) includes machine-generated radiation such as from accelerated particles and from radioacti- ve isotopes that have been artificially produced. In diagnostic applications, γ rays are of major interest as they consist of high-energy photons with a mass of zero resulting in less interactions with the target material (e.g. tissue), and therefore high penetration through the whole body. While cell death in tumors or inflamed tissue is desired in therapeutic nuclear medi- cine [9, 10], in diagnostic nuclear medicine and diagnostic radiology the dose should be kept to a minimum to prevent these effects. Hence for therapeutic and diagnostic application of radiation in humans, radiation dosimetry is necessary to control the 1 Hereinafter referred to as ”dose”. 2 In the scientific literature there is controversy concerning the correct model which accounts for the stochastic effects at low doses [6]. Most regulatory bodies rely on the linear-no-threshold (LNT) model which assumes that even the smallest doses increase cancer risk. However, the LNT model lacks infor- mation at low doses where a linear fit function was created from higher dose rate data (collected from nuclear bomb survivors). Hence, the extrapolation was done beyond the limits of observed data.[7, 8] 2 chapter 1. Introduction dose (see 1.3) absorbed by tissues, organs and systems of organs with respect to the extend of a desired effect or the probability of a (stochastic) effect, respectively. This thesis is based on the diagnostic application of radioligands (radiation emit- ting substances bound to an active biomolecule) after intravenous injection. These compounds are called radiotracers. This concept is based on the pioneering work of George Hevesy [11, 12]. It describes a radiotracer as a chemical compound in which one or more atoms have been replaced by one of its radioactive isotopes. The injec- ted amount of radiotracer will not interfere with the processes which are studied, as the concentrations used are extremely low as compared to the concentrations that would cause pharmacological effects [11, 13]. Thus, in vivo measurement including imaging of several different targets improves the early diagnosis for example of neu- rodegenerative diseases [14, 15], schizophrenia [16] and cancer [17]. Furthermore, it may support the development of new drugs by target evaluation, drug efficiency stu- dies and contribute to the understanding of complex biochemical processes [18, 13]. At the University Hospital Leipzig, brain disorders are routinely investigated with positron emission tomography (PET). Neuropathological studies indicated that al- terations of physiological metabolic processes in the brain may set on before the age of 30 years in patients who develop neurodegenerative disease [15, 19, 20]. Hence, different tracers e.g. targeting proteins or specific receptors were developed aiming at an early diagnosis of those diseases [15, 21, 22, 23, 24, 25]. At the research site Leipzig of the Helmholtz-Zentrum Dresden-Rossendorf two promising radiotracers 18 were developed, [ F]flubatine [26, 27, 28] for PET-imaging of α4β2 nicotinic acetyl- 18 choline receptors and [ F]fluspidine [29] for the imaging of σ1 receptors in several brain disease such as depression and neurodegeneration. The preclinical and clini- cal radiation dosimetry of the four radiotracers (-)-[18F]flubatine, (+)-[18F]flubatine, (S)-(-)-[18F]fluspidine and (R)-(+)-[18F]fluspidine are presented and discussed in this thesis. The summary in chapter 3 is based on the three publications Sattler3 et al. (2014)[30], Kranz et al. (2016a)[31] and Kranz et al. (2016b)[32]. 1.1 Radiation dosimetry One part of radiation physics deals with the investigation of the mean absorbed energy from the interaction of ionizing radiation with matter while radiation dosi- metry is the quantitative determination of that energy [33]. There are two types of voluntary or involuntary exposure of humans to ionizing ra- diation, external or internal, which require particular dosimetric considerations. In 3 Bernhard Sattler and Mathias Kranz are equally contributing first authors. 1.1. Radiation dosimetry 3 medical applications of ionizing radiation in humans, both approaches are used: (i) external dosimetry in X-ray applications like computed tomography (CT), brachy- therapy applications or external beam radiation therapy and (ii) internal dosimetry in radionuclide therapy or diagnostic imaging with radionuclides in nuclear medici- ne for instance planar/emission computed tomography -scintigraphic applications. External dosimetry describes the dose in humans if the radiation source is outside of the body. Hence, with types of radiation or accelerated particles that penetrate the skin (beta, photon or neutron radiation). In turn, internal dosimetry is the mea- surement of the dose caused by the ionizing radiation from radioactive substances that have entered the body (e.g. via ingestion, inhalation or injection).[34, 35] Internal and external dosimetry observes the mean absorbed dose (statistically averaged) in the respective volume disregarding the inherent random fluctuations. Hence, the macroscopic effects are objective for this type of investigation. Another field of dosimetry is referred to as microdosimetry. It observes the energy deposition in cellular and sub-cellular structures where fluctuations are known to have large effects to these small volumes.[36] This thesis is based on internal (macroscopic) dosimetry of voluntary intravenous application of radioligands for visualization of brain functions with PET. To meet the regulatory requirements of the competent German authority (section 1.1.3) for newly developed radioligands applied first time in human, the radiation safety has to be proven in preclinical studies. Therefore, different steps need to be followed to achieve dosimetry results in animals that yield to represent a dose estimate for humans, like (i) choosing the right investigational protocol so it fits the subsequent human study, (ii) quantitatively exact PET measurement, (iii) data reconstruction and image registration, (iv) collection of the time-dependent radioactivity concen- tration data (biodistribution), (v) conversion and extrapolation of the data to the respective human scales, (vi) calculation of the
Details
-
File Typepdf
-
Upload Time-
-
Content LanguagesEnglish
-
Upload UserAnonymous/Not logged-in
-
File Pages79 Page
-
File Size-