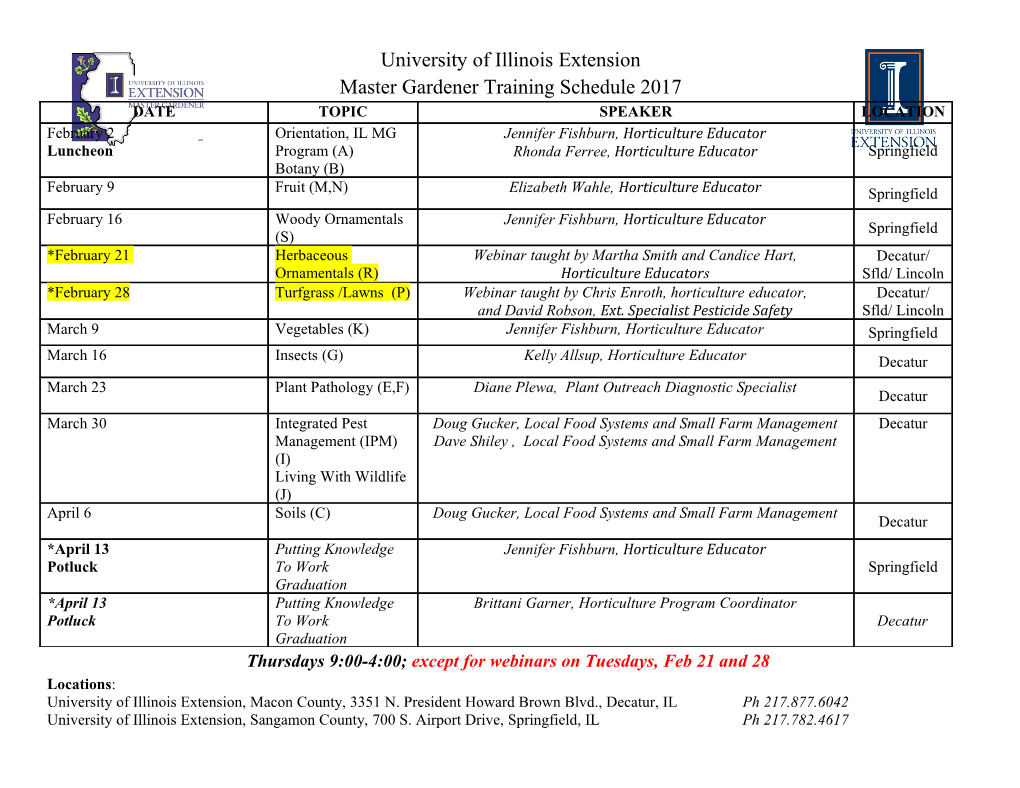
International Journal of Biochemistry and Cell Biology 112 (2019) 1–7 Contents lists available at ScienceDirect International Journal of Biochemistry and Cell Biology journal homepage: www.elsevier.com/locate/biocel Medicine in focus Extracellular regulation of airway smooth muscle contraction T ⁎ Fatemeh Khadangi, Ynuk Bossé Laval University, Canada ARTICLE INFO ABSTRACT Keywords: The molecular mechanisms governing the contraction of airway smooth muscle have always been at the fore- Airway hyperresponsiveness front of asthma research. New extracellular molecules affecting the contraction of airway smooth muscle are Bronchodilators steadily being discovered. Although interesting, this is disconcerting for researchers trying to find a mend for the Spasmogens significant part of asthma symptoms caused by contraction. Additional efforts are being deployed to understand the intracellular signaling pathways leading to contraction. The goal being to find common pathways that are essential to convey the contractile signal emanating from any single or combination of extracellular molecules. Not only these pathways exist and their details are being slowly unveiled, but some carry the signal inside-out to interact back with extracellular molecules. These latter represent targets with promising therapeutic potential, not only because they are molecules downstream of pathways essential for contraction but also because their extracellular location makes them readily accessible by inhaled drugs. 1. Introduction 2. Pathogenesis Airway smooth muscle (ASM) is a tissue for which the physiological Asthma is a lung disorder characterizes by variable airflow ob- function is uncertain but that contributes significantly to respiratory struction. The symptoms include wheezing, thoracic oppression, re- symptoms in asthma. Understanding the molecular mechanisms reg- spiratory distress and cough. One of the most objective criteria to di- ulating the contraction of ASM is thus of utmost importance. agnose asthma is the presence of reversible airway obstruction, which is The first section of this article describes the uncontested role of ASM defined as an increase in forced expiratory volume in 1 s (FEV1) of more in asthma pathogenesis. The second section reviews the newly identi- than 200 ml and greater than 12% of the predicted value following the fied extracellular molecules and receptors capable of contracting, re- inhalation of a drug that relaxes the ASM (i.e., a bronchodilator such as laxing or modulating ASM contraction. The third section expands on salbutamol). This feature testifies that ASM contributes to asthma recently discovered contractile signaling pathways in the intracellular symptoms. However, the assortment of extracellular molecules leading space that link back to components in the extracellular space. Finally, to excessive ASM contraction in asthma is complex, heterogenous and the fourth section briefly discusses about the therapeutic potential of unstable. This not only means that a great number of molecules may these latest breakthroughs. contribute to ASM contraction in each patient but that the molecules involved are likely to be variable between patients, as well as to change Abbreviations: Abi1, Abl interactor 1; ADAM33, A disintegrin and metalloproteinase 33; AHR, airway hyperresponsiveness; ArfGAPs, ADP-ribosylation factor GTPase-activating proteins; Arp2/3, Actin-related proteins 2/3; ASM, airway smooth muscle; BPIFA1, Bacterial permeability family member A1; Ca2+, calcium; c- Abl, Abelson tyrosine kinase; CAS, Crk-associated substrates; CaSR, Ca2+-sensing receptor; Cdc42GAP, Cdc42 GTPase-activating protein; CTTN, Cortactin; DOCK, Dedicator of cytokinesis; DPA, docosahexaenoic acid; ECM, extracellular matrix; ELMO, Engulfment and cell motility protein; FAK, Focal adhesion kinase; F-actin, actin filament; FEV1, forced expiratory volume in 1 second; FFAR1, Free fatty acid receptor 1; G-actin, globular (monomeric) actin; GEF, guanine nucleotide exchange factor; GIT, G-protein-coupled receptor kinase-interacting protein; GMF-γ, Glia maturation factor-; GPCR, G protein-couple receptor; GTPase, guanosine tripho- sphatase; HDAC8, Histone deacetylase 8; IL, interleukin; ILK, Integrin-linked kinase; Mfge8, Milk fat globule-EGF factor 8; MLC, Myosin light chain; N-WASp, Neuronal Wiskott-Aldrich syndrome protein; NMII, Non-muscle myosin II; NMHCII, Non-muscle myosin II heavy chain; NM MLC, Non-muscle myosin light chain; Pak, p21-activated kinase; PIP2, phosphatidylinositol 4,5-biphosphate; PIP5K1, Phosphatidylinositol 4-phosphate 5-kinase 1; PINCH, Particularly interesting new cysteine-histidine-rich protein; PIX, Pak-interacting exchange factor; Plk1, Polo-like kinase 1; Pfn-1, Profilin-1; PP1, Protein phosphatase 1; SLK, Ste20-like kinase; ROCK, Rho-associated coiled-coil containing protein kinase; SSAT, Spermidine/spermine N-acetyltransferase; TMEM16A, Transmembrane protein 16A; TNFα, Tumor necrosis factor; VASP, Vasodilator-stimulated phosphoprotein; WTN-5A, Wingless-integrase-1 family member 5A ⁎ Corresponding author at: IUCPQ, Pavillon Mallet, M2694, 2725, chemin Sainte-Foy, Québec, Qc, G1V 4G5, Canada. E-mail address: [email protected] (Y. Bossé). https://doi.org/10.1016/j.biocel.2019.04.012 Received 8 February 2019; Received in revised form 25 April 2019; Accepted 26 April 2019 Available online 28 April 2019 1357-2725/ © 2019 Elsevier Ltd. All rights reserved. F. Khadangi and Y. Bossé International Journal of Biochemistry and Cell Biology 112 (2019) 1–7 over time within each patient. asthma (Hanania et al., 2016; Russell et al., 2018). Similar claims can be made for TNF-α (Wenzel et al., 2009) and Interleukin-17 (IL-17) 3. Extracellular molecules controlling ASM contraction (Busse et al., 2013). Targeting a single molecule in asthma is certainly appealing and compatible with the emerging spirit of promoting pre- The search for extracellular molecules affecting the contraction of cision medicine to address the well-recognized molecular heterogeneity ASM is very active. Overall, hundreds of molecules are directly or in- of asthma pathogenesis between patients. The drawback is that, beyond directly involved (Bosse, 2012). Apart from the classical bronchodila- inter-individual variability, there is complexity. The complexity sig- tors (e.g., prostaglandin E2 and nitric oxide) and spasmogens (e.g., nifies that within any given affected individual, many molecular defects acetylcholine and histamine), new molecules affecting ASM contraction may be contributing. Blocking only one of them is thus expected to are steadily being discovered. Due to space constraint, this article can benefit, at most, very little (Chang and Bosse, 2016). only report on few recent examples. The Bacterial permeability family member A1 (BPIFA), an anti- 4. Inside-out signal required for ASM contraction microbial protein secreted by the airway epithelium, attenuates ASM contraction (Wu et al., 2017). Resolvin D2, a D-series resolvin derived Additional efforts are directed towards the understanding of the from the ω-3 docosahexaenoic acid, prevents the development of ASM intracellular pathways leading to ASM contraction. The goal is to hypercontractility elicited by either leukotriene D4 and Tumor necrosis identify pathways for which targeting would block contraction irre- factor α (TNFα)(Khaddaj-Mallat et al., 2016). Leptin, a hormone spective of the dysregulated bronchoactive molecules involved. controlling energy intake, acts through the cholinergic neurons in the Conveniently, it turns out that the contractile signals downstream of central nervous system to decrease parasympathetic efferences and the many cell-surface receptors do converge into common intracellular release of acetylcholine in the lungs, thereby alleviating muscarinic 3 pathways that are essential for contraction. A typical example is the receptor-mediated ASM contraction (Arteaga-Solis et al., 2013). phosphorylation of the Myosin light chain (MLC) and the subsequent S100A8 also decreases ASM contraction (Xu et al., 2017). Contrastingly, stimulation of the actin-activated ATPase on the myosin head. These the epithelium-derived Neuropeptide Y (Li et al., 2016c), Wingless-in- downstream steps enable the chemical energy contained in ATP to be tegrase-1 family member 5 A (WTN-5 A) (Koopmans et al., 2016) and transformed into mechanical force and shortening as the myosin heads high concentration of carbon dioxide (i.e., hypercapnia) (Shigemura bound on actin filaments strive to pivot successively, in a process called et al., 2018) promote airway hyperresponsiveness (AHR) by increasing cross-bridge cycling, to pull the actin filaments. the contractile capacity of ASM. Ironically, recent discoveries in the field of intracellular signaling Many receptors were also recently shown to influence ASM con- bring back to the fore the importance of signaling towards the extra- traction. Toll-like receptor-7, an intracellular receptor that specifically cellular space (Fig. 1). Indeed, some intracellular pathways that are recognizes single-stranded bacterial and viral RNAs, relaxes ASM in- essential for contraction elicited by any spasmogen lead to the re- directly by stimulating the synthesis of nitric oxide from the nerves arrangement of the cell’s cytoskeleton in order to reinforce the con- (Drake et al., 2013). The ionotropic N-methyl-D-aspartate receptors nection of the contractile apparatus inside the cell with the extracellular either contract ASM directly in healthy conditions or relax ASM in- matrix (ECM)
Details
-
File Typepdf
-
Upload Time-
-
Content LanguagesEnglish
-
Upload UserAnonymous/Not logged-in
-
File Pages7 Page
-
File Size-