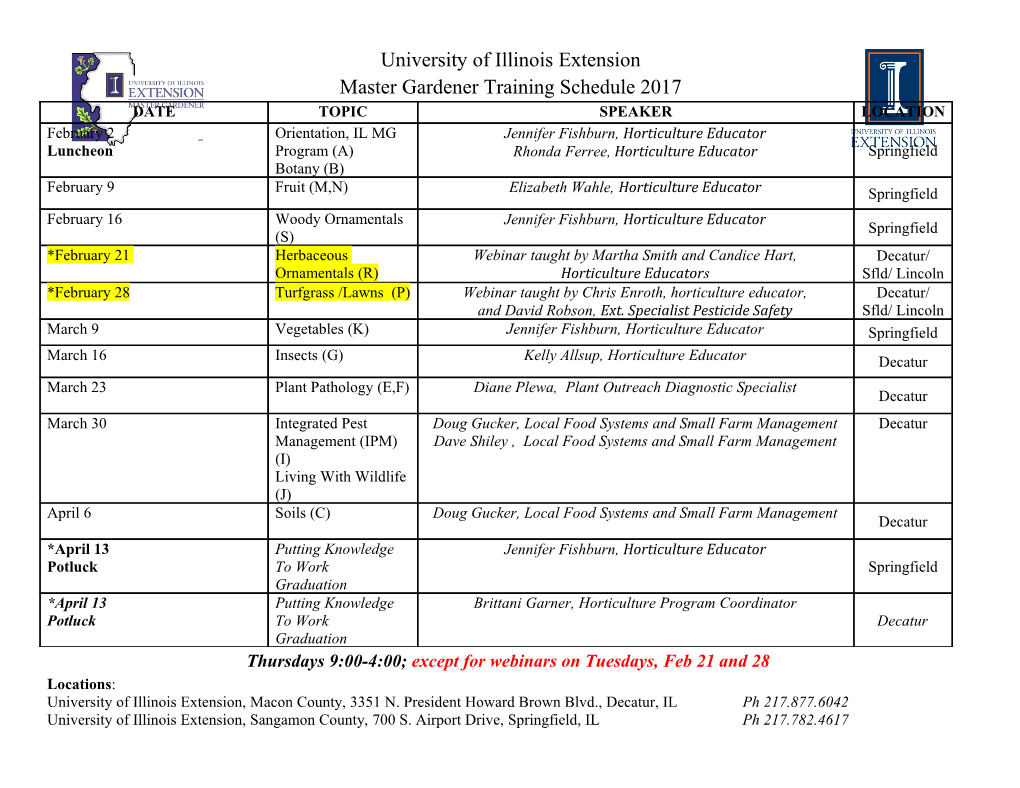
Open Journal of Veterinary Medicine, 2016, 6, 40-58 Published Online February 2016 in SciRes. http://www.scirp.org/journal/ojvm http://dx.doi.org/10.4236/ojvm.2016.62006 A Review of Enrofloxacin for Veterinary Use Tessa Trouchon, Sébastien Lefebvre USC 1233 INRA-Vetagro Sup, Veterinary School of Lyon, Marcy l’Etoile, France Received 12 January 2016; accepted 21 February 2016; published 26 February 2016 Copyright © 2016 by authors and Scientific Research Publishing Inc. This work is licensed under the Creative Commons Attribution International License (CC BY). http://creativecommons.org/licenses/by/4.0/ Abstract This review outlines the current knowledge on the use of enrofloxacin in veterinary medicine from biochemical mechanisms to the use in the field conditions and even resistance and ecotoxic- ity. The basics of biochemistry, the mechanisms of action and resistance and pharmacokinetics are presented. Then an overview of available veterinary products, their efficacy and their toxicity against target species, human and environment is provided. Keywords Enrofloxacin, Antibiotic Resistances, Veterinary 1. Introduction Enrofloxacin (Figure 1(c)), or 1-Cyclopropyl-6-fluoro-7-(4-ethyl-1-piperazinyl)-1,4-dihydro-4-oxo-3-quinoli- necarboxylic acid, belongs to fluoroquinolone family which is a subfamily of quinolone. The first quinolone is the Nalidixic acid (Figure 1(a)) used in animal at the beginning of 1980s, enrofloxacin is the first fluoroquino- lone patented in 1984 [1]. The huge evolution in the quinolone family is the addition of a fluor atom on the 6th position which improves quinolones’ antibacterial spectrum [2] and creates the fluoroquinolone subfamily. Quinolones have an action on bacterial topoisomerase. The marketing authorization reports a large antimicrobial spectrum for enrofloxacin, which is efficient on most gram-negative and gram-positive bacteria but not efficient on anaerobic bacteria [3]. But with 3.6 tons sold per year in France for animal use [4], fluroroquinolones are an important family in veterinary medicine that increases the probability of selecting resisting bacteria. 2. Action Mechanism of Enrofloxacin 2.1. Important Physicochemical Properties of Enrofloxacin Enrofloxacin is a zwitterionic molecule with a pKa1 = [5.88 - 6.06] and a pKa2 = [7.70 - 7.74] [5]. The lowest How to cite this paper: Trouchon, T. and Lefebvre, S. (2016) A Review of Enrofloxacin for Veterinary Use. Open Journal of Veterinary Medicine, 6, 40-58. http://dx.doi.org/10.4236/ojvm.2016.62006 T. Trouchon, S. Lefebvre Figure 1. Structure of (a) Nalidixic Acid; (b) Ciprofloxacin and (c) Enrofloxacin. pKa is due to the carboxyl acid group and the second to the basic tertiary amine. So enrofloxacin doesn’t bear charge between this two pH. Moreover it is a lipophilic molecule with a logP of 4.70 at a pH of 7 [6], but 1.88 ± 1.43 with ACDLabs. The Active metabolite of enrofloxacin, ciprofloxacin (Figure 1(b)) or 1-Cyclopropyl-6-fluoro-1, 4-dihydro-7- (1-piperazinyl)-4-oxo-3-quinoline carboxylic acid, which is available as a drug product on veterinary and human market, is a multiple acid with pKa1 = 5.15 and pKa2 = 8.25 [7]. Ciprofloxacin is less lipophilic than enroflox- acin with a logP of −1.11 at a pH of 7.4 [8]. 2.2. Targets’ Mechanism of Action Enrofloxacin, like the other quinolones, has two mains targets of the topoisomerase family. Although these pro- teins exist in eukaryotes cells, quinolones have less affinity for eukaryotes’ topoisomerases than for the DNA Topoisomerase II (Gyrase) and the DNA Topoisomerase IV (Topo IV) two major bacterial topoisomerase [9]. The Gyrase and the Topo IV are two tetramers (Figure 2(a)) formed respectively of two GyrA and two GyrB and of two ParC and two ParE. Moreover GyrA and GyrB are homologous respectively with ParC and ParE [10]. The Gyrase has an important role in bacteria’s life by modifying the topology of the spiral DNA. Indeed, the positive supercoiling stabilizes the DNA and the strands’ separation becomes more difficult [11]. Moreover transcription generates a positive supercoiling accumulation that can stop transcription. This positive supercoil- ing can be released by the Gyrase, which enhances transcription [12]. To do this, the Gyrase binds and wraps around itself a strand of DNA (Figure 2(b)) with the help of the C-terminal domain [13] and cleaves it (Figure 2(c)) with mediating of the catalytic tyrosine Tyr 122 [14] of each GyrA. Tyrosines form covalent phosphotyro- syls with each 5’ phosphoryl terminus of both strands, which remain binding during the reaction [15]. This reac- tion forms a gap in sequence (called G-DNA). The GyrB part catches the other DNA sequence (called trans- ported DNA or T-DNA). The T-DNA is passed through the opened G-DNA (Figure 2(d)) [16]. The G-DNA is closed in an ATP-dependent reaction (Figure 2(e)) [17]. Of this reaction results an adding of negative coiling. The Gyrase is able to do an intermolecular strand passage at the end of the replication like the Topo IV but 41 T. Trouchon, S. Lefebvre Figure 2. Mechanism of action of Gyrase, with intervention of Gyrase part A (GyrA) and B (GyrB), gap DNA sequence (G-DNA), transported DNA sequence (T-DNA), ATP and GDP. (a) Gyrase with GyrA dimer and GyrB dimer; (b) Interaction of Gyrase with two DNA sequences; (c) Fixation of an ATP molecule and cleavage of the G-DNA strand; (d) Passage of the T-DNA strand through G-DNA; (e) Closing of the G-DNA strand with ATP degradation. without wrapping the DNA as the Topo IV, this is the major difference between reactions of both enzymes [18]. Topo IV catalyzes the segregation of the two daughter DNA molecules after the replication [19] more efficiently than Gyrase [20]. 2.3. Interactions between Fluoroquinolones, DNA and Its Target The penetration in the bacteria differs between Gram-positives and Gram-negatives bacteria. For Gram-negative bacteria, fluoroquinolones pass the outer membrane mainly through porins [21] [22]. The trimeric OmpF porin is mainly used by fluoroquinolone, this pathway can be modulated by Mg2+ [23]. But it seems that some qui- nolones can promote a pathway by interacting with the Lipopolysaccharide (LPS) and create a lipophilic passage [24]. For Gram-positive bacteria the diffusion process is the main uptake pathway [25]. In the case of mycobacteria the high lipid level of the membrane allows fluoroquinolones to diffuse through membrane [26]. After the entrance in the bacteria, quinolones have two effects on the bacteria: bacteriostatic at low concentra- tion level or bactericide at high concentration level. Rapidly after the Gyrase has formed a complex with the DNA strand, two quinolones bind with this complex, before the DNA’s cleavage [27]. This binding is reversible and induces a conformation modification in Gyrase [28]. This modification induces a cleavage of DNA in a par- ticular location and forms a cleaved complex [29] [30]. At this step the action is still reversible, but reduces rap- idly the activity of replication and has a bacteriostatic effect [31]. The next two pathways are possible one for bacteriostatic concentration and the other for bactericide concen- tration. For bacteriostatic concentrations, quinolones induce the SOS regulon controlled by the repressor lexA [32] [33]. Activation of the regulon induces the sfiA gene which codes for an inhibitor of cell division and bac- teria form a long filamentous structure. This effect is still reversible [34]. This filamentous structure contributes to the death of bacteria [35]. 42 T. Trouchon, S. Lefebvre For bactericide concentration quinolones induce a chromosome fragmentation by creation of a suicide factor or by destabilization of a GyrA dimer [35]. The fragmentation can induce a rapid death or, by reassociation of the GyrA dimer, genomic mutation in bacteria [36]. 2.4. Resistance Mechanism The resistance to fluoroquinolones in general, is today a main subject for the use of antibiotic substances in vet- erinary medicine and was the subject of an EMA report [4]. Many studies show an augmentation in the devel- opment of resistances to enrofloxacin [37] [38]. Moreover, another study shows the increase of resistance of Escherichia coli to quinolones and fluoroquinolones after using of enrofloxacin in calves [39]. The mechanism of resistance to fluoroquinolones is based on many pathways: the change of targets, the pro- tection of target, the lower expression of target, the improvement of the efflux pumps, and inactivation of fluoroquinolones. These mechanisms can be chromosomal or plasmide-mediated (Table 1) [40]. The most common mechanism is the mutation of Gyrase. In the subpart GyrA the mutation is mainly between Ala67 and Gln 106 for E.coli and other bacteria [41] [42]. The mutation is near the GyrA active site which is called Quinolone Resistance-Determining Region (QRDR). For GyrB two mutation are reported at Asp426 and Lys447 [43]. Only one Gyrase mutation is able to increase the Minimum Inhibitory Concentration (MIC) up to 64 times [41], but the GyrB mutation seems to be less effective in resistance against nalidixic acid than the GyrA mutation [44]. The mutation of Topo IV is also described but, until today, always with at least one Gyrase mutation. There- fore, these mutations seem to be developed in a second time to increase the resistance more than only Gyrase’s mutations. A QRDR can be also characterized in the parC of topoisomerase IV between Tyr 57 and Glu84 [45]. The protection of target can be attributed to a qnr gene often present on a Plasmide-Mediated Quinolone Re- sistance (PMQR) or in the chromosomal DNA. The Qnr protein is a dimere which binds with Gyrase and de- creases the binding of quinolones with DNA-Gyrase-Qnr complex. But it seems that the Qnr protein reduces the interaction between DNA and Gyrase [46].
Details
-
File Typepdf
-
Upload Time-
-
Content LanguagesEnglish
-
Upload UserAnonymous/Not logged-in
-
File Pages19 Page
-
File Size-