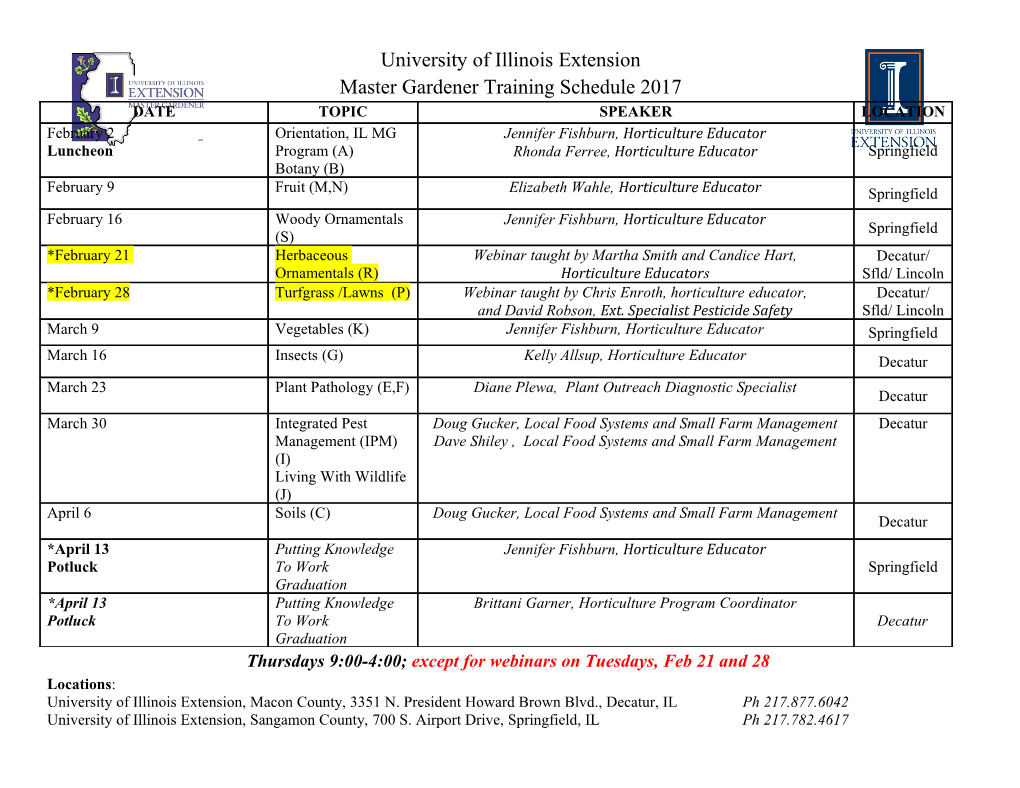
Environmental Microbiology (2017) 19(8), 3132–3151 doi:10.1111/1462-2920.13788 Functional structure of the bromeliad tank microbiome is strongly shaped by local geochemical conditions Stilianos Louca,1,2* Saulo M. S. Jacques,3,4 denitrification steps, ammonification, sulfate respira- Aliny P. F. Pires,3 Juliana S. Leal,3,5 tion, methanogenesis, reductive acetogenesis and 6 1,2,7 Angelica L. Gonzalez, Michael Doebeli and anoxygenic phototrophy. Overall, CO2 reducers domi- Vinicius F. Farjalla3 nated in abundance over sulfate reducers, and 1Biodiversity Research Centre, University of British anoxygenic phototrophs largely outnumbered oxy- Columbia, Vancouver, BC, Canada. genic photoautotrophs. Functional community 2Department of Zoology, University of British Columbia, structure correlated strongly with environmental vari- Vancouver, BC, Canada. ables, between and within a single bromeliad species. 3Department of Ecology, Biology Institute, Universidade Methanogens and reductive acetogens correlated Federal do Rio de Janeiro, Rio de Janeiro, Brazil. with detrital volume and canopy coverage, and exhib- 4Programa de Pos-Graduac ¸ao~ em Ecologia e ited higher relative abundances in N. cruenta.A Evoluc¸ao,~ Universidade Estadual do Rio de Janeiro, Rio comparison of bromeliads to freshwater lake sedi- de Janeiro, Brazil. ments and soil from around the world, revealed stark differences in terms of taxonomic as well as func- 5Programa de Pos-Graduac ¸ao~ em Ecologia, tional microbial community structure. Universidade Federal do Rio de Janeiro, Rio de Janeiro, Brazil. 6Biology Department & Center for Computational & Introduction Integrative Biology, Rutgers University, Camden, NJ, USA. Bromeliads (fam. Bromeliaceae) are plants found through- 7Department of Mathematics, University of British out the neotropics, with many species having rosette-like Columbia, Vancouver, BC, Canada. foliages that can accumulate water and detritus (e.g. dead leaves from the surrounding canopy) in a central cavity (Kitching, 2001). The accumulation and decomposition of Summary detritus inside these cavities (‘tanks’) provides nutrients to Phytotelmata in tank-forming Bromeliaceae plants are the plant (Benzing and Renfrow, 1974; Endres and Mer- regarded as potential miniature models for aquatic cier, 2003; Romero et al., 2006) and to a plethora of ecology, but detailed investigations of their microbial organisms residing in the tanks (Srivastava, 2006). In communities are rare. Hence, the biogeochemistry in coastal sand dune forests or ‘restingas’ (Fig. 1A), which bromeliad tanks remains poorly understood. Here we cover 70% of the Brazilian coast, bromeliads can reach 21 investigate the structure of bacterial and archaeal densities over 15 000 ha and constitute moist and communities inhabiting the detritus within the tanks nutrient-rich oases amid a predominantly sandy and of two bromeliad species, Aechmea nudicaulis and nutrient-poor environment (Cogliatti-Carvalho et al., 2001). Neoregelia cruenta, from a Brazilian sand dune forest. In such environments, bromeliad tanks are biodiversity hot- We used metagenomic sequencing for functional spots and central hubs for biomass and regional nutrient community profiling and 16S sequencing for taxo- cycling (Rocha et al., 2000; Romero et al., 2006; Martinson nomic profiling. We estimated the correlation et al., 2010). A great number of organisms, including bac- between functional groups and various environmen- teria, archaea, fungi, protozoans, insects, amphibians and tal variables, and compared communities between reptiles, spend part or all of their life cycle in these tanks bromeliad species. In all bromeliads, microbial com- (Rocha et al., 2000). These organisms can engage in a munities spanned a metabolic network adapted to plethora of interactions and ecosystem processes includ- oxygen-limited conditions, including all ing active nitrogen fixation (Brighigna et al., 1992; Goffredi et al., 2011b; Giongo et al., 2013), photosynthesis (Bouard Received 19 October, 2016; accepted 24 April, 2017. *For Corre- et al., 2012), chemolithotrophy (Goffredi et al., 2011a), her- spondence. E-mail [email protected] bivory, predation and detritivory (Ngai and Srivastava, VC 2017 Society for Applied Microbiology and John Wiley & Sons Ltd Functional structure of the bromeliad tank microbiome 3133 A B Aechmea nudicaulis C Neoregelia cruenta D 10 cm 10 cm light intensity number plant tank detrital tank temperature salinity shading (%) ( mol/m /s) of leaves height (cm) depth (cm) volume (mL) volume (mL) pH (PSU) EFGHP=0.006 P=0.40 P<0.001 P<0.001 I P<0.001 J P=0.43 KLP<0.001 P=0.04 MP=0.86 N P=0.01 turbidity water color DOC oxygen (NTU) (1/m) TSS (mg/L) conc. (mg/L) total N ( M) total P ( M) molar N:P CH4 ( M) N2O ( M) (% satur.) OPQRSP=0.11 P=0.006 P=0.04 P<0.001 P=0.94 T P=0.84 U P=0.70 V P=0.099 W P=0.03 X P=0.78 A.n. N.c. A.n. N.c. A.n. N.c. A.n. N.c. A.n. N.c. A.n. N.c. A.n. N.c. A.n. N.c. A.n. N.c. A.n. N.c. Fig. 1. Sampling site and bromeliads. A. Sampling site in the Jurubatiba National Park, on the east coast of Brazil. B. Aechmea nudicaulis (also indicated by white arrows in A) and (C) Neoregelia cruenta, the two bromeliad species considered in this study and the dominant bromeliad species in Jurubatiba. The rosette-like foliage arrangement forms multiple small peripheral cavities and a larger central tank, which accumulate rainwater, litter and dead animals and support intense nutrient cycling by specialized microbial communities. D. Detritus extracted from the bottom of a bromeliad tank. E–X. Box-whisker plots of physical (E–L) and chemical (M–X) variables across 22 A. nudicaulis (A.n., red) and 9 N. cruenta (N.c., blue) bromeliads. Whiskers show 95% percentiles around the medians. Statistical significances of differences in mean values between A. nudicaulis and N. cruenta (P-values based on permutation tests) are indicated in the plots. [Colour figure can be viewed at wileyonlinelibrary.com] 2006). Extensive research on protist and animal communi- gradient gel electrophoresis (DGGE) revealed rich and ties in bromeliad tanks reveals a food web structure that is highly variable microbial communities without, however, comparable in complexity to much larger aquatic ecosys- providing detailed information on taxonomic or functional tems (Richardson, 1999; Kitching, 2000; Rocha et al., structure (Farjalla et al., 2012). Sequencing of 16S ribo- 2000; Marino et al., 2013). Because bromeliad tanks con- somal RNA (rRNA) clone libraries (Goffredi et al., 2011b) stitute semi-isolated and self-contained systems, they are and shotgun environmental mRNA sequencing (Goffredi considered ideal models for ecosystem ecology, biogeog- et al., 2015) have provided further insight on microbial tax- raphy and climate change research (Srivastava et al., onomic composition and on active metabolic pathways in 2004; Farjalla et al., 2012; Atwood et al., 2013; Brandt rainforest bromeliads. These studies revealed that decom- et al., 2015). position of organic matter leads to oxygen depletion inside The bulk of organic matter decomposition and nutrient bromeliad tanks, where oxygen (O2) can drop below micro- cycling within bromeliad tanks is catalysed by microorgan- molar levels within the detrital mass accumulating at the isms (Bouard et al., 2012). Using bromeliad tanks as tank bottom (Guimaraes-Souza et al., 2006). This oxygen models for ecosystem ecology thus requires a solid under- depletion promotes fermentative activity that can decrease standing of the microbial component and the biochemical pH to levels below 4 (Goffredi et al., 2011b). Along such 2 22 processes that it catalyses. The vast majority of past stud- strong redox gradients, nitrate (NO3 ), sulfate (SO4 )and ies on bromeliad tank communities either exclusively eventually carbon dioxide (CO2) may become alternative focused on animals or only considered microbial commu- terminal electron acceptors for bacterial and archaeal res- nity structure at coarse resolutions, for example, piration (Canfield and Thamdrup, 2009), potentially combining all bacteria into a single group (Ngai and Srivas- causing nitrogen loss via denitrification or driving sulfur tava, 2006; Farjalla et al., 2012; Marino et al., 2013; cycling and methanogenesis. High concentrations of Gonzalez et al., 2014). Previous work utilizing denaturation methanogens and intense methanogenesis have indeed VC 2017 Society for Applied Microbiology and John Wiley & Sons Ltd, Environmental Microbiology, 19, 3132–3151 3134 S. Louca et al. been detected in bromeliads (Martinson et al., 2010; Gof- study, we examine this functional composition in detail and fredi et al., 2011a; Brandt et al., 2016), however the role of discuss its meaning in terms of the biogeochemistry of the other potentially important anaerobic pathways remains bromeliad tank ecosystem. To facilitate the interpretation of unknown. Dissimilatory sulfur and nitrogen metabolism, for metagenomic content, whenever possible, we associated example, are typically active in other aquatic sediments detected taxa with various potential metabolic functions and oxygen-depleted water columns, where they strongly using the existing literature on cultured organisms (Louca shape local physicochemical conditions (Canfield and et al., 2016b,a). To explore the potential interaction Thamdrup, 2009). Moreover, the relative roles of various between environmental conditions and functional commu- microbial functional groups, including photoautotrophs, nity structure, we performed
Details
-
File Typepdf
-
Upload Time-
-
Content LanguagesEnglish
-
Upload UserAnonymous/Not logged-in
-
File Pages20 Page
-
File Size-