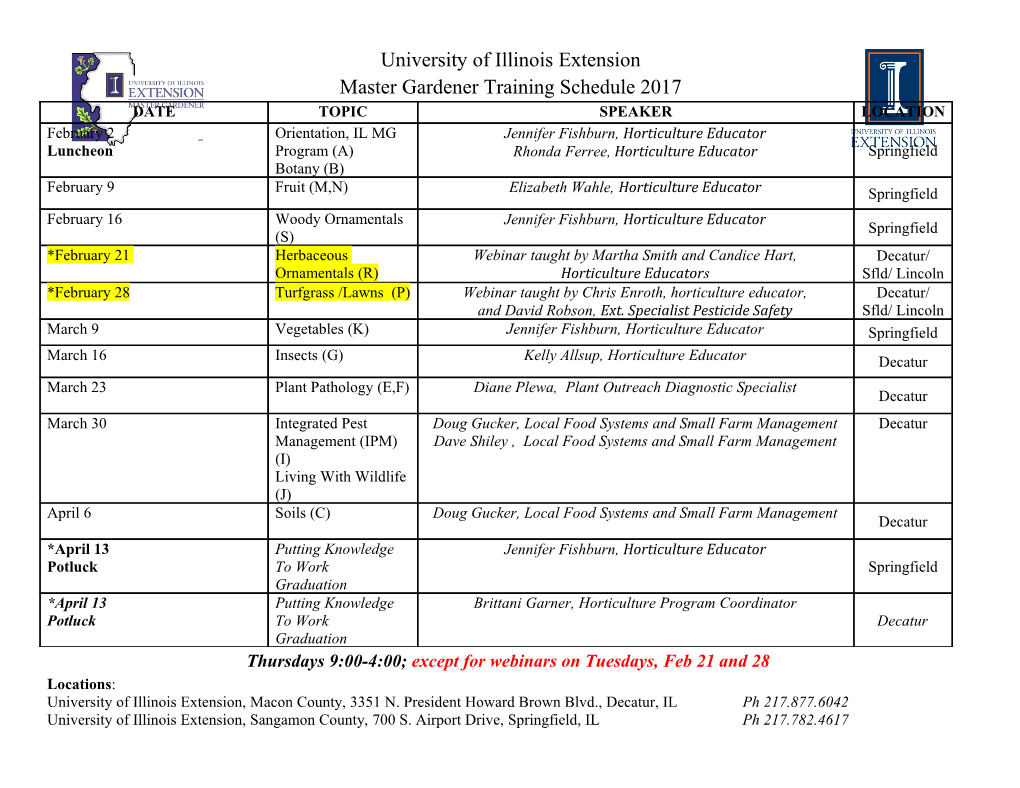
12.10 Climate Regulation as a Service from Estuarine and Coastal Ecosystems S Heckbert and R Costanza, Portland State University, Portland, OR, USA ES Poloczanska and AJ Richardson, CSIRO Marine and Atmospheric Research, Brisbane, QLD, Australia © 2011 Elsevier Inc. All rights reserved. 12.10.1 Introduction 200 12.10.1.1 Definition and Global Occurrence 200 12.10.1.2 Climate Regulation Services 201 12.10.1.3 Anthropogenic Impacts on Climate Regulation Services from Estuaries and Coasts 201 12.10.2 Climate Regulation Services of Coastal Habitats 202 12.10.2.1 Salt Marshes 203 12.10.2.2 Mangroves 204 12.10.2.3 Seagrass Meadows 205 12.10.2.4 Kelp Forests 206 12.10.2.5 Coral Reefs 207 12.10.2.6 Estuaries 207 12.10.2.7 Continental Shelf Seas 208 12.10.3 Coastal Anthropogenic Impacts and Changes in GHG Dynamics 209 12.10.3.1 Coastal Modification and Use 209 12.10.3.2 Impacts of Climate Change 210 12.10.4 Maintaining ES and Mitigating GHGs in Coastal Zones 210 12.10.4.1 Adaptation and Mitigation Potential 210 12.10.5 Conclusions 212 References 213 Abstract Coastal regions, at the interface between terrestrial and oceanic ecosystems, play an important role in global biogeochemical cycles. This chapter reviews the climate regulation services of estuarine and coastal ecosystems (ECEs) including tidal salt marshes, mangroves, seagrass beds, macroalgal forests, coral reefs, and coastal shelf ecosystems. ECEs regulate global and regional climates by sequestering or releasing carbon dioxide and other greenhouse gases (GHGs). ECEs are extremely productive biologically, with net primary production rates per unit area among the highest of any ecosystem. Consequently, ECEs play a globally significant role as carbon sinks, with carbon storage rates per unit area of many habitats far exceeding that of land habitats at the rate of about 10 times that of temperate terrestrial forests and 50 times that of tropical forests. Furthermore, sedimentation does not reach an equilibrium carbon balance as occurs in terrestrial systems, whose sequestration capacity is forecasted to decrease this century. Conversely, they are large potential sources of GHG's if disturbed or misman­ aged. Critically, carbon sequestration in many coastal habitats is superior to that of terrestrial habitats, as carbon is generally stored over long time frames (thousands of years) as a consequence of the large belowground biomass and the absence of fire threat. Furthermore, carbon is generally broken down anaerobically; hence, emissions of other potent GHGs such as methane and nitrous oxide are negligible. A review of literature provided sequestration rates for various coastal habitats. Using these in combination with global extent of selected habitats, this chapter finds that GHGs worldwide, mangroves, seagrass beds, and salt marshes combine to sequester a minimum of 136 000 tonnes C annually into long-term carbon storage. Assuming prices of CO2e from $10 to $90 per tonne, the value of the annual sequestration is $5 –45 billion. This is an underestimate due to data gaps and, the limited assessment of the area of these three coastal ecosystem habitats, and relates only to long-term storage. The figures do not include short-term carbon storage in biomass, and further unaccounted for carbon sequestration occurs in kelp forests, estuaries, and coastal shelf seas. Many ECEs are under threat globally from sea-level rise, coastal development, pollution, and other anthropogenic stressors, and protection and restoration of ECEs may be an important tool for mitigating climate change. Currently, these habitats are not included in the United Nations Framework Convention on Climate Change (UNFCCC) carbon accounting frameworks, and therefore are excluded from incentive schemes such as carbon markets and other incentive programs, but their inclusion deserves consideration due to their potential for mitigating global climate change. The global distribution of C sequestration in ECEs reveals that large areas of the tropics are home to the highest sequestration rates and occur in developing countries, which also have the highest rates of coastal habitat degradation. Schemes such as Reducing Emissions from Deforestation and Forest Degradation (REDD) may bring revenues and 199 200 Climate Regulation as a Service from Estuarine and Coastal Ecosystems added benefits to developing countries for instigating projects and marine protected areas for conservation. Many small island nations and developing countries in the tropics are particularly vulnerable to climate change and contain large swathes of seagrasses and mangroves compared to overall land area, but do not contain large areas of forests or grasslands, which would apply to REDD in its current form. Coastal habitats thus not only present an untapped potential for inclusion in climate change mitigation schemes, but also present a little-recognized risk of loss of large carbon stocks if their degradation and destruction are not reduced or halted. 12.10.1 Introduction Mitigation possibilities include protecting ECEs through con­ servation initiatives, thereby maintaining existing stocks and C Coastal regions, at the interface of terrestrial and oceanic ecosys­ sequestration rates. Restoration projects for degraded ECEs can tems, play an important role in global biogeochemical cycles of renew their ability to sequester C (Erwin, 2009). the biosphere. Estuarine and coastal ecosystems (ECEs) are cap­ However, regulations and market-based instruments are not able of regulating both global and regional climates by yet present to incentivize climate change mitigation projects in sequestering or releasing carbon and other greenhouse gases ECEs. For terrestrial ecosystems, various mechanisms exist to (GHGs), and by modifying local temperature and evapotran­ financially support GHG mitigation projects, such as carbon spiration regimes. They are extremely productive biologically, markets, statutory incentive schemes of national governments, with net primary production (NPP) rates per unit area among and international initiatives such as Reducing Emissions from the highest of any ecosystem. They are generally heterotrophic Deforestation and Forest Degradation (REDD). Terrestrial eco­ systems, largely due to the inputs of nutrients and sediments systems have accepted GHG accounting frameworks and from terrestrial systems via rivers and tidal flows, and thus UNFCCC guidelines for project-level accounting, and require release carbon dioxide and methane through biotic respiration. assurances on the additionality and permanence of C seques­ Sediments in seagrass and tidal wetland habitats tend to be tered. However, accounting frameworks for ECEs are not yet oxygen deficient; hence, soil respiration and the release of car­ robust compared to terrestrial ecosystems and, therefore, mea­ bon from decaying matter by microbes and fungi are repressed, suring the change in carbon stocks resulting from coastal and thus bioaccumulation of organic matter is larger than in mitigation projects is insufficient for assuring financial incen­ terrestrial ecosystems. In addition, mangroves, salt-marsh plants, tives. Extending existing protection measures such as marine and seagrasses have considerable production below ground in protected areas can conserve ecosystem functions. We thus find roots and rhizomes, which enhances belowground accumula­ that coastal habitats present an untapped potential for inclusion tion of carbon. This coastal vegetation is estimated to sequester in climate change mitigation schemes such as REDD, particularly carbon at the rate of about 10 times that of temperate terrestrial for developing countries of the tropics where vast extents of these forests and 50 times that of tropical forests (Pidgeon, 2009). coastal habitats are found. However, disturbance of ECEs also Furthermore, sequestration in coastal systems through sedimen­ presents a little-recognized risk of releasing existing C stocks, if tation does not reach an equilibrium carbon balance as occurs in their degradation and destruction are not reduced or halted. terrestrial systems, whose sequestration capacity is forecasted to decrease this century (Friedlingstein et al., 2006; Phillips et al., 12.10.1.1 Definition and Global Occurrence 2008; Piao et al., 2009). ECEs therefore represent large potential carbon sinks, yet they are also large potential sources of GHGs, if Coastlines vary greatly around the world from frozen polar disturbed or mismanaged. This chapter reviews the climate reg­ shorelines to tropical mangroves and beaches. There is esti­ ulation services of key components of ECEs, including tidal mated to be >1.6 million km of coastline globally (UNEP, marshes, mangroves, seagrass beds, macroalgal forests, coral 2006). Coastal populations are growing rapidly, with over a reefs, and coastal shelf seas. third of the world’s population living on or near the coast, and Impacts on ECEs include conversion or degradation from more than half of the coastal countries presently having >80% anthropogenic activities and also climate change. of their population living in coastal areas (Burke et al., 2001; Anthropogenic activities include deforestation of mangroves, UNEP, 2006; Martinez et al., 2007). Here, we define the coastal and destruction of seagrass meadows through sedimentation region to include coastal seas on the continental shelf (to a from land catchment runoff, fishing practices, and coastal depth of 200 m), the coastline, and the adjacent land that is development. Water quality
Details
-
File Typepdf
-
Upload Time-
-
Content LanguagesEnglish
-
Upload UserAnonymous/Not logged-in
-
File Pages18 Page
-
File Size-