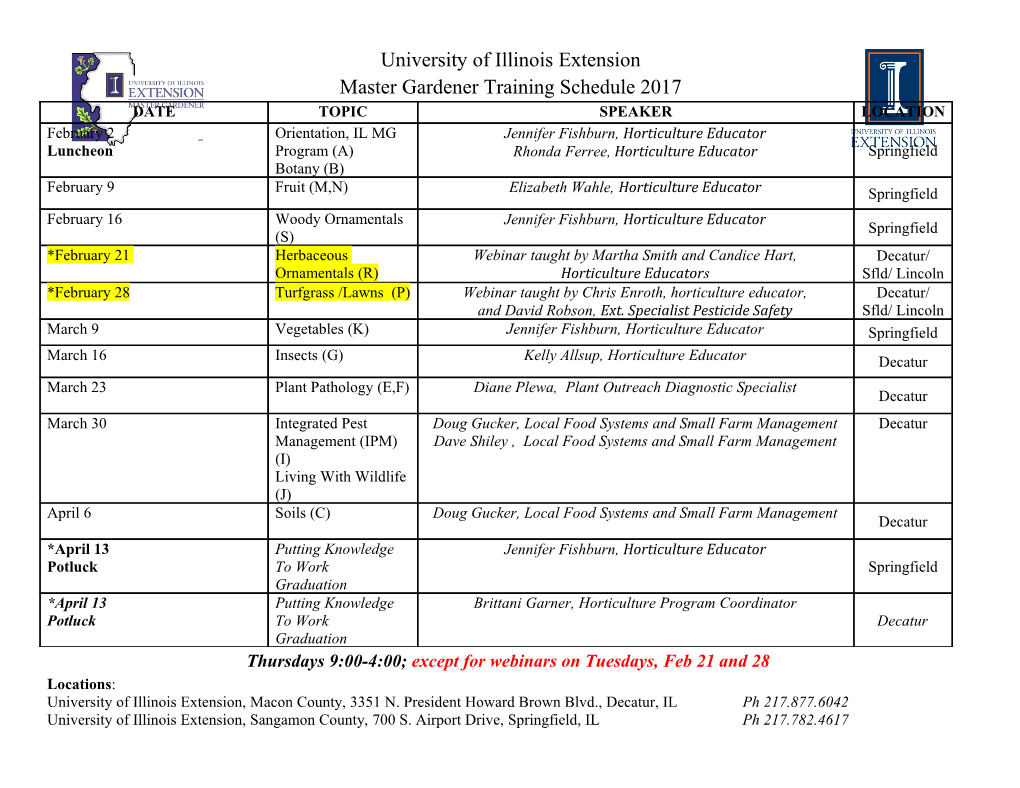
https://doi.org/10.1130/G48687.1 Manuscript received 10 April 2020 Revised manuscript received 2 December 2020 Manuscript accepted 16 January 2021 © 2021 The Authors. Gold Open Access: This paper is published under the terms of the CC-BY license. Published online 22 March 2021 Spatio-temporal patterns of Pyrenean exhumation revealed by inverse thermo-kinematic modeling of a large thermochronologic data set Magdalena Ellis Curry1, Peter van der Beek2, Ritske S. Huismans3, Sebastian G. Wolf3, Charlotte Fillon4 and Josep-Anton Muñoz5 1 Department of Earth and Atmospheric Sciences, University of Houston, Houston, Texas 77204, USA 2 Institute for Geosciences, University of Potsdam, 14469 Potsdam, Germany 3 Department of Earth Science, University of Bergen, 5020 Bergen, Norway 4 TOTAL EP, R&D Nexts, 64018 Pau, France 5 Geomodels Research Institute, University of Barcelona, 08007 Barcelona, Spain ABSTRACT data set (n = 264) and incorporate topographic Large thermochronologic data sets enable orogen-scale investigations into spatio-temporal boundary constraints to evaluate controls on patterns of erosion and deformation. We present the results of a thermo-kinematic model- orogen-scale exhumation in the Pyrenees. We ing study that examines large-scale controls on spatio-temporal variations in exhumation aim to explain two key features of the data: (1) as recorded by multiple low-temperature thermochronometers in the Pyrenees mountains an along-strike trend in exhumation, and (2) the (France/Spain). Using 264 compiled cooling ages spanning ∼200 km of the orogen, a recent existence of post-orogenic cooling ages. Our model for its topographic evolution, and the thermo-kinematic modeling code Pecube, we results show that spatio-temporal patterns of evaluated two models for Axial Zone (AZ) exhumation: (1) thrust sheet–controlled (north- exhumation are controlled by structural inheri- south) exhumation, and (2) along-strike (east-west) variable exhumation. We also measured tance and post-orogenic surface processes, and the degree to which spatially variable post-orogenic erosion influenced the cooling ages. We to a limited extent, by thrust-sheet kinematics. found the best fit for a model of along-strike variable exhumation. In the eastern AZ, rock uplift rates peak at ≥1 mm/yr between 40 and 30 Ma, whereas in the western AZ, they peak TECTONIC CONTEXT between 30 and 20 Ma. The amount of post-orogenic (<20 Ma) erosion increases from <1.0 km The Pyrenean orogen formed due to Late in the eastern Pyrenees to >2.5 km in the west. The data reveal a pattern of exhumation that Cretaceous to Miocene convergence between is primarily controlled by structural inheritance, with ancillary patterns reflecting growth Europe and Iberia. Prior to convergence, both and erosion of the antiformal stack and post-orogenic surface processes. the Paleozoic Variscan orogeny and Mesozoic extension affected the region (e.g., Muñoz, INTRODUCTION ally, a recently published model of orogen-scale 2019). Structural inheritance from this tectonic The feedback between tectonically driven topographic evolution (Curry et al., 2019) con- history persists in the modern orogen, with Pyre- rock trajectories and erosion controls orogenic strains surface uplift through time, providing a nean thrusts exploiting a now-inverted Mesozoic topography, generates exhumation, and inte- rare opportunity to investigate spatio-temporal extensional fault system, producing the domi- grates crustal deformation, lithology, surface variations in both exhumation and rock uplift. nant east-west structural trend (Fig. 1). The processes, mantle dynamics, and climate (e.g., Pyrenean exhumation is variably attributed mountain belt is divided into two oppositely Whipple, 2009; Jamieson and Beaumont, 2013). to (1) southward-propagating deformation of an verging thrust belts. The Southern Pyrenees Thermochronology is the prime tool for quanti- orogenic wedge (Fitzgerald et al., 1999; Sinclair thrust belt makes up the pro-wedge (downgo- fying exhumation and, when paired with inde- et al., 2005), (2) westward-propagating defor- ing plate) and is further divided into the Axial pendent constraints on topography, can provide mation due to variable pre-orogenic crustal Zone (AZ) and the South Pyrenean zone (SPZ; valuable information for investigating these rela- extension (e.g., Puigdefàbregas et al., 1992; Fig. 1). The AZ comprises an antiformal stack tionships. A large spatial- and temporal-scale Whitchurch et al., 2011), and/or (3) base-level of Paleozoic basement thrust sheets in the east- approach facilitates investigation into the rates, dynamics (Coney et al., 1996; Fillon and van ern and central Pyrenees (Muñoz, 1992; Ver- patterns, and drivers of orogen-wide exhumation der Beek, 2012). The localized nature of most gés et al., 2002). The SPZ is a south-vergent within a regionally consistent framework. The Pyrenean thermochronologic studies prevents thin-skinned fold-thrust belt that propagates into Pyrenees mountains (France/Spain) present a validation of a consistent model of exhuma- the pro-foreland Ebro Basin (Fig. 1). The Ebro unique study area because they comprise a small tion; while several studies examine across-strike Basin became endorheic in the late Eocene, and orogen with a well-constrained tectono-sedi- trends, few investigate along-strike variation. >2 km of conglomeratic material was deposited mentary evolution, for which spatially extensive We use inverse thermo-kinematic modeling of and later excavated when the basin reopened in thermochronologic data are available. Addition- a large, compiled multi-thermochronometer the Miocene (Coney et al., 1996). The North CITATION: Curry, M.E., et al., 2021, Spatio-temporal patterns of Pyrenean exhumation revealed by inverse thermo-kinematic modeling of a large thermochrono- logic data set: Geology, v. 49, p. 738–742, https://doi.org/10.1130/G48687.1 738 www.gsapubs.org | Volume 49 | Number 6 | GEOLOGY | Geological Society of America Downloaded from http://pubs.geoscienceworld.org/gsa/geology/article-pdf/49/6/738/5323312/g48687.1.pdf by guest on 27 September 2021 A STUDY AREA Aquitaine Basin NPZ 43 N° Neogene/Quaternary Cenozoic Sierras Exteriores AXIAL Mesozoic Figure 1. (A) Pyrenees N study area geology. ZONE Paleozoic basement, plutonic massifs ECORS—Etude Conti- nentale et Océanique 50 km Sample location par Réflexion et Réfrac- SPZ tion Sismique seismic survey. (B) Model topog- Sierras ECORS raphy from Curry et al. Marginales 42 N° (2019); gray envelope is Ebro Basin uncertainty. (C,D) Model setups. NPZ, SPZ—North, 0° 1 E° 2 E° 3° South Pyrenean zone, B 2 Model topography evolution C Model A D Model B respectively. NPF—North 43° 43° Pyrenean fault. NPF NPZ NPF NPZ 1.5 Lakhora zone Present Nogueres zone ZONE 1 1 42.5° topography 42.5° ZONE 2 Orri zone Nogueres Mean elevation (km) 0.5 zone 50 40 30 20 10 0 ZONE 3 ZONE 4 Time (Ma) 42° 0° 1° 2° 42° 0° 1° 2° Pyrenean zone (NPZ) is a north-verging thick- into four independent zones distributed east- track (AFT, n = 123), zircon (U-Th)/He (ZHe, skinned belt of inverted Mesozoic basins that west along strike (Fig. 1D). We treat the NPZ n = 40), and zircon fission-track (ZFT, n = 24) makes up the retro-wedge (Muñoz, 2019). as distinct from the AZ due to its earlier exhu- data (Fig. 2) (Morris et al., 1998; Fitzgerald mation history (Fitzgerald et al., 1999; Vacherat et al., 1999; Sinclair et al., 2005; Gibson et al., DATA AND METHODS et al., 2016). 2007; Jolivet et al., 2007; Maurel et al., 2008; We used the thermo-kinematic modeling We invert for (1) rock-uplift rate through Gunnell et al., 2009; Metcalf et al., 2009; Her- code Pecube (Braun, 2003; Braun et al., 2012) to time, and (2) thickness of overburden depos- man et al., 2013; Bosch et al., 2016; Vacherat track rock-particle paths and temperatures dur- ited and subsequently removed in the Miocene et al., 2016; Fillon et al., 2020). These data ing exhumation and to predict thermochronom- (Fig. S1). In each inversion, the orogenic period collectively record rock cooling from ∼300 to eter ages given an imposed tectonic (rock-uplift) (56–20 Ma) was divided into seven 5 m.y. time ∼60 °C and encompass the pre-, syn-, and post- and topographic (surface-uplift) scenario. We bins (earliest bin is 6 m.y. to coincide with the orogenic periods. Ages vary from ca. 170 Ma used an inversion approach to identify a tectonic beginning of the Eocene at 56 Ma) to identify for unreset ZFT in the NPZ to ca. 6 Ma for scenario within a predefined parameter space potential changes in rock-uplift rate (Fig. S1); AHe in the western AZ; the bulk of the ages that best fits the available thermochronometer for each time bin, the model inverts for the uplift are between ca. 50 and ca. 20 Ma. The AFT data (details are provided in the Supplemental rate that best reproduces the thermochronology and ZFT ages are single-sample, multi-grain Material1). Each inversion consists of 10,080 data. The post-orogenic (<20 Ma) uplift rate “central ages”; the AHe and ZHe ages are either forward models that converge on a combina- was set at 0.01 km/m.y., following previous aliquot or average single-grain ages. All sample tion of input parameters resulting in the lowest inversions (Fillon and van der Beek, 2012). ages, uncertainties, locations, and references are calculated χ2 misfit. We then assessed the inver- The models include surface uplift (topographic given in Table S2. sion results by calculating the marginal poste- evolution) based on the results of Curry et al. rior probability density function of each inverted (2019), which involves topographic growth from SPATIO-TEMPORAL PATTERNS OF parameter, thus providing a Bayesian estimate 56 to 23 Ma and minor decay from 23 to 0 Ma EXHUMATION of parameter resolution (cf. Braun et al., 2012). (Fig. 1B). Including independently determined Model B (along-strike variable uplift; Fig.
Details
-
File Typepdf
-
Upload Time-
-
Content LanguagesEnglish
-
Upload UserAnonymous/Not logged-in
-
File Pages5 Page
-
File Size-