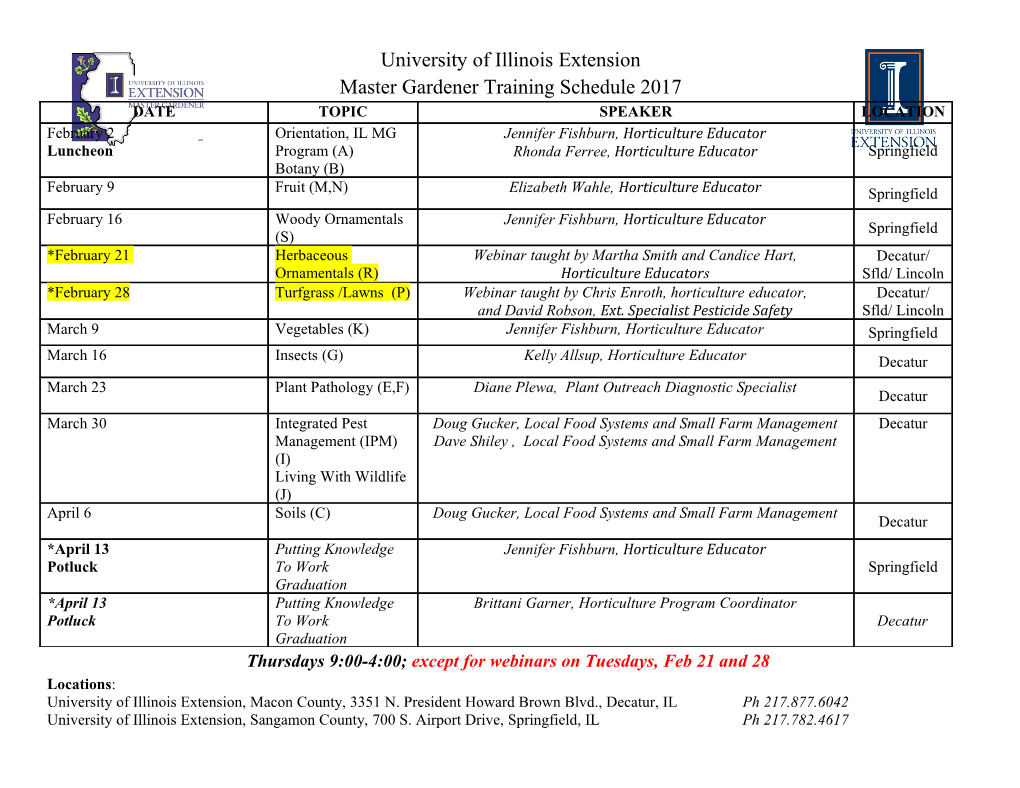
OPIOID PATHWAYS IN METHAMPHETAMINE INTAKE BY EMILY C. EASTWOOD A DISSERTATION Presented to the Department of Behavioral Neuroscience and the Oregon Health & Science University School of Medicine in Partial Fulfillment of the Requirements for the Degree of Doctor of Philosophy April 2015 OREGON HEALTH & SCIENCE UNIVERSITY SCHOOL OF MEDICINE – GRADUATE STUDIES School of Medicine Oregon Health & Science University CERTIFICATE OF APPROVAL ___________________________________ This is to certify that the PhD dissertation of Emily C. Eastwood has been approved ______________________________________ Tamara J Phillips, PhD (member/advisor) ______________________________________ John Crabbe, PhD (member) ______________________________________ Mary Heinricher, PhD (member) ______________________________________ Aaron Janowsky, PhD (member) __________________________________ John Belknap, PhD (member) TABLE OF CONTENTS LIST OF FIGURES iv LIST OF TABLES vi LIST OF ABBREVIATIONS vii ACKNOWLEDGEMENTS x ABSTRACT xii CHAPTER 1: General Introduction 1 Methamphetamine History and Abuse Epidemiology 1 Methamphetamine Pharmacokinetics and Pharmacology 2 Mechanisms of methamphetamine action 4 Direct Actions of Methamphetamine 4 Monoamine transporters 4 Indirect Actions of Methamphetamine 5 y-aminobutryic Acid and Glutamate 5 Opioids and Opioid Receptors 7 Physiological and Subjective Effects of Methamphetamine 9 Methods for Examining Rewarding and Aversive Effects of Drugs in Mice 12 Two-bottle Choice Drinking 12 Operant Models 14 Conditioned Place Preference and Conditioned Place Aversion 15 Conditioned Taste Aversion 18 Methods for the Investigation of Genetic Influences on Drug Effects 19 Quantitative Trait Locus Mapping 19 Standard Inbred and Recombinant Inbred Strains 22 Single Gene Manipulations 25 i Selectively Bred Lines 27 Gene Expression 32 Genetic Risk for Human Methamphetamine Use 34 Pharmacological Treatment of Methamphetamine Abuse 36 Dopamine-Transporter and Receptor Targeting Drugs 37 Serotonin Receptor Targeting Drugs 39 y-aminobutryic Acid Receptor Targeting Drugs 39 Opioid-Receptor Targeting Drugs 39 MA Effects in MOP-r Genetically Modified Mice 40 Selected Lines for MA-related Phenotypes 41 Genetic Factor Influencing MA Intake in Selectively Bred Mice 43 Experimental Goals and Hypotheses 45 CHAPTER 2: Opioid Sensitivity in Mice with Either High or Low Genetic 48 Susceptibility to Methamphetamine Intake CHAPTER 3: Methamphetamine Drinking Microstructure in Mice Bred to Drink High or Low Amounts of Methamphetamine 75 CHAPTER 4: Opioid Intake and Effects of Mu-opioid Receptor Drugs on Methamphetamine Intake 102 CHAPTER 5: Opioid Treatment to Reduce Oral Methamphetamine Intake and Verification of a Methamphetamine Intake QTL 128 CHAPTER 6: General Discussion 165 Differences in sensitivity to and avidity for MOP-r agonist drugs 167 Opioid pharmacokinetics in MADR mice 171 MOP-r binding in MADR and B6 and D2 mice 171 MA drinking microstructure and blood levels in MADR mice 174 Pharmacological manipulations of MA drinking 176 ii Verification and finer mapping of a QTL for MA intake 183 Summary and Conclusions 186 Future Directions 186 REFERENCES 188 iii LIST OF FIGURES Figure 1.1: Circuit diagram depicting the neuronal connections superimposed 5 on the approximate anatomical locations of each brain region. Figure 2.1: Sensitivity to the Analgesic Effects of Fentanyl in MADR Mice 58 Using the Hot Plate and Tail Flick Tests. Figure 2.2: Sensitivity to the Analgesic Effects of Fentanyl in MADR Mice 60 Using the Magnesium Sulfate-Induced Abdominal Writhing Test. Figure 2.3: Sensitivity of MADR Mice to the Locomotor Stimulant Effects 62 of Fentanyl. Figure 2.4: Sensitivity of MADR Mice to the Locomotor Stimulant Effects 64 of Morphine. Figure 2.5: Consumption of MA in D2 and B6 Mice in a Two-Bottle 66 Choice Drinking Procedure. Figure 2.6: Morphine Clearance between MADR-2 Mice. 68 Figure 2.7: Fentanyl Clearance between MADR-2 Mice. 70 Figure 3.1 Consumption of morphine and quinine in MADR mice, when offered as 87 alternative solutions in a two-bottle choice study. Figure 3.2: Consumption of morphine by MADR mice in a saccharin fading study 89 Figure 3.3: Pretreatment with Naltrexone does not Alter MA drinking in MADR 91 Mice. Figure 3.4: Pretreatment with Higher doses of Naltrexone does not Alter MA 93 Drinking in MADR Mice. Figure 3.5: Low Doses of Buprenorphine Reduce MA Intake. 96 Figure 4.1: Consumption of MA and water in MADR mice, when offered in a 110 4-h limited access two-bottle choice study. Figure 4.2: Temporal pattern of licks taken from the MA-containing bottle. 112 Figure 4.3: MA drinking pattern characteristics. 114 iv Figure 4.4: MA intake across the first 4 days of MA access. 116 Figure 4.5: MA drinking pattern characteristics across the first 4 days of MA 118 access. Figure 3.6: MA Consumption and Corresponding Blood MA levels in 121 MAHDR-2 and MALDR-2 Mice. Figure 5.1: Diagram representing the genotype of the Chr 10 D2.B6 0-7.72 and 135 D2.B6 0-20.4 congenic strains. Figure 5.2: Higher Doses of Morphine Reduce MA Intake 142 Figure 5.3: High Doses of Morphine Reduce Saccharin Intake 146 Figure 5.4: Consumption of MA and total volume during a 4h limited access 149 two-bottle drinking session in a lickometer apparatus. Figure 5.5: Consumption of MA and total volume during a 4h limited access 152 two-bottle drinking session in a lickometer apparatus. Figure 5.6: A high dose of FENT reduced licks from the MA and water-containing 153 bottle during the first 30 min of a 4 h session. Figure 5.7: MA Drinking in Chr 10 D2.B6 (0-7.72) and Chr 10 D2.B6 (0-20.4) 157 congenic and D2 mice. Figure 6.1: Revised Circuit diagram depicting the neuronal connections 182 superimposed on the approximate anatomical locations of each brain region. v LIST OF TABLES Table 3.1: Schedule of fluids offered during morphine vs. quinine two-bottle 80 choice drinking study Table 3.2: Schedule of fluids offered during saccharin fading two-bottle choice 82 study Table 5.1: Effects of morphine on total volume consumed during a MA vs water 144 two-bottle choice drinking procedure. Table 5.2: Total volume consumed during a saccharin vs water two-bottle 147 choice drinking procedure. Table 5.3: MOP-r density and affinity in MADR, B6 and D2 mice. 157 Table 6.1: Summary of differences in MOP-r agonist drug sensitivity 166 Table 6.2: Summary of the opioid pharmacokinetic and binding data from 170 MADR, B6 and D2 mice Table 6.3: Summary of MA microstructure and corresponding MA 173 blood levels in MADR mice. Table 6.4: Summary of the effects of MOP-r drugs on MA intake in MADR mice 175 vi LIST OF ABBREVIATIONS ADHD – attention deficit hyperactivity disorder AMPA - a-amino-3-hydroxy-5-methyl-4-isoazolepropionic acid AMPH – amphetamine BC - backcross BUP – buprenorphine B6 – C57BL6/J Chr - chromosome CNS – central nervous system CPA – conditioned place aversion CPP- conditioned place preference CS+ - positive conditioned stimulus CTA - conditioned taste aversion DA – dopamine DAT – dopamine transporter DE – differentially expressed D2 – DBA/2J ERK – extracellular signal regulated kinase ES – embryonic stem FENT - fentanyl GABA – y-aminobutyric acid GAD – glutamic acid decarboxylase GLU – glutamate GWAS – genome wide association study HCl – hydrochloride vii HMACT - high methamphetamine activation HSP – heat shock protein IL - infralimbic IP - intraperitoneal IV – intravenous LMACT – low methamphetamine activation LOD – log of the odds MA – methamphetamine MADR – methamphetamine drinking MAHDR – methamphetamine high drinking MAHSENS – methamphetamine high sensitization MALDR – methamphetamine low drinking MALSENS – methamphetamine low sensitization MOR - morphine mPFC – medial prefrontal cortex MOP-r – Mu-opioid receptor NAc – nucleus accumbens NC - nociceptin NE - norepinephrine NET – norepinephrine transporter NIDA – National Institute on Drug Abuse NMDA - N-methyl-D-aspartate ORL-1 – opioid receptor like 1 PFC – prefrontal cortex PKC – protein kinase C PL - prelimbic viii PPD - preprodynorphin PPE – preproenkephalin qPCR – quantitative polymerase chain reaction QTL – quantitative trait locus RI – recombinant inbred RIST – recombinant inbred segregation test SERT – serotonin transporter SENS - sentization SNc – substantia nigra pars compacta SNP – single nucleotide polymorhphism SNr – substantia nigra pars reticulata SSRI – selective serotonin reuptake inhibitor US – unconditioned stimulus UTR – untranslated region VMAT2 – vesicular monoamine transporter 2 VMb – ventral midbrain VTA – ventral tegmental area ix ACKNOWLEDGEMENTS There are a number of people that I would like to express my deepest gratitude to for influencing my decision to attend graduate school and to complete my doctoral research. First and foremost, I would like to thank my graduate school advisor, Dr. Tamara Phillips. Throughout the years she has taught me; to humbly accept criticism, how to critically think, and has molded me into a rigorous scientist. She has provided invaluable training in data analysis and statistical design. Without her, the last 6 years would not have been possible. I also would like to recognize my undergraduate Professor, Dr. Lauren Liets, who influenced my interest in attending graduate school. During my undergraduate education at UC Davis, I took two courses from her; Advanced Systemic Physiology and Neurobiology of Addictive Drugs. The first course piqued my interest in research and the second introduced me to this field of research. I would also like to thank
Details
-
File Typepdf
-
Upload Time-
-
Content LanguagesEnglish
-
Upload UserAnonymous/Not logged-in
-
File Pages246 Page
-
File Size-