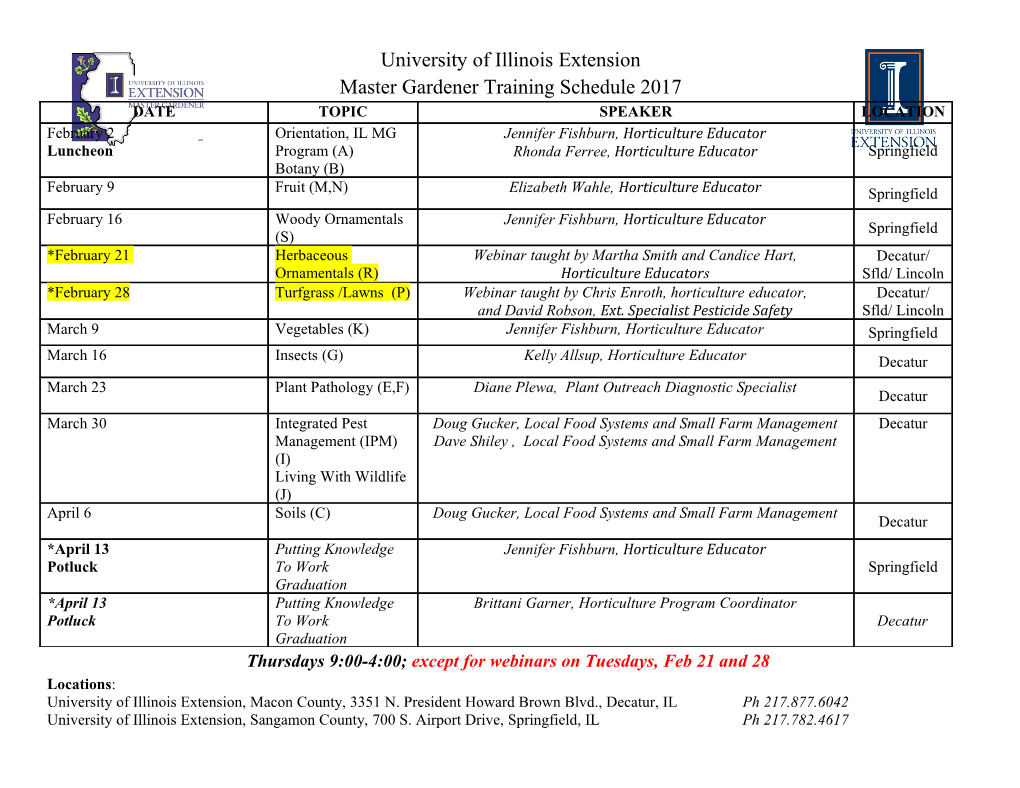
Radiation trapping in a cold atomic gas Guillaume Labeyrie,1 Robin Kaiser,1, ∗ and Dominique Delande2 1Institut Non Lin´eaire de Nice, 1361 route des Lucioles, F-06560 Valbonne, France. 2Laboratoire Kastler Brossel, Universit´e Pierre et Marie Curie, 4 Place Jussieu, F-75005 Paris, France (Dated: September 16, 2005) We experimentally study radiation trapping of near resonant light in a cloud of laser cooled rubidium atoms. Unlike in most previous studies, dealing with hot vapors, collisional braodening is here negligible and Doppler broadening due to the residual atomic velocity is narrower than the homogenous broadening. This is an interesting new regime, at the boundary between coherent and incoherent radiation transport. We analyze in detail our low temperature data (quasi elastic regime), then provide some experimental evidence for Doppler-based frequency redistribution. The data are compared with an analytical model valid for coherent transport and a Monte-Carlo simulation including the Doppler effect. PACS numbers: 42.25.Dd, 32.80.Pj I. INTRODUCTION ples of large optical thicknesses. A convenient mean to observe this is to study radiation trapping (RT). Trap- Ultra-cold atomic vapors have recently come to the ping of resonant radiation in atomic vapors has been the attention of researchers in the field of mesoscopic focus of numerous studies dating back to the beginning physics [1]. Indeed, cold atoms can be manipulated with of the 20th century. Holstein used in 1947 an hypoth- a high degree of control and mesoscopic samples with esis of complete frequency redistribution (CFR) at each unprecedented purity and tunability can be assembled. scattering event – where the frequency of the outgoing An emerging field of study deals with the transport of photon is completely uncorrelated with the frequency of near resonant light in such media. Using cold atoms, one the incoming photon – to derive decay rates in various can realize a monodisperse ensemble of strongly resonant situations [4]. Since then, many theoretical approaches point scatterers, free of defects and absorption, where beyond CFR where employed to deal with experimental the light mean free path can be varied continuously, sim- observations (see e.g. [5–10]). With the advent of laser ply by tuning the laser frequency without manipulation cooling in the eighties, new experimental regimes became of the sample. These properties are very attractive to accessible towards the coherent propagation of light in study localization of light in strongly scattering media. atomic gases. However, it was only at the end of the 20th However, coherent transport requires that the light re- century that studies of light transport in optically thick mains monochromatic, which is possible only if scatter- clouds of cold atoms started to appear [11, 12]. In a pre- ing is fully elastic. In the limit of low saturation of the vious paper [12], we focussed on transport in the quasi- atomic transition (e.g., at low light intensity for resonant elastic regime and showed that, owing to the strongly res- light), the scattering by an atom at rest can be consid- onant character of atomic scatterers, the transport veloc- ered essentially elastic and no frequency redistribution is ity which characterizes the propagation of energy in the present. However, even at low temperature, the Doppler diffuse light was more than 4 orders of magnitude smaller effect on the slowly moving atoms makes the frequency than the vacuum speed of light. In the present work, of the scattered photon to be slightly different from the we discuss in more details the role of frequency redis- frequency of the incoming photon. Moreover, this fre- tribution in laser-cooled vapors, which offers the unique quency change depends on the relative orientations of opportunity to study the transition from coherent to in- the atomic velocity and the incoming and outgoing wave coherent transport. A typical coherent effect is the ex- vectors of the photons. Altogether, some frequency re- istence of a speckle pattern when light is scattered by a distribution occurs because of the residual atomic mo- disordered medium with frozen disorder. Although this tion (Doppler effect). For an atomic vapor which is has not been observed with atomic clouds in the multi- laser cooled to approximately 80µK, the Doppler broad- ple scattering regime, it is likely to exist if the atoms are ening is only a small fraction of the natural (homoge- sufficiently cold (coherent transport regime). With not- nous) linewidth. Frequency redistribution is thus small, so-cold atoms, the scattered light has a relatively broad which indeed allows to observe interferential effects in the frequency distribution and interference effects responsi- multiple scattering regime such as coherent backscatter- ble for speckle are likely to be washed out (incoherent ing [2, 3]. However, redistribution may become impor- transport). Another well-known interference effect is co- tant if many scattering events are chained i.e., for sam- herent backscattering, which is observed [13] to be quite sensitive to the residual atomic velocity. We first give in section II a very brief description of the principles of the experiment and numerical simulation. We then ∗email:[email protected] present in section III experimental data in the regime × −2 SCP below 5 10 , which means that the scattering process is mainly elastic. Also, with a probe pulse duration of typ- diffuse ically a few µs, less than ten photons are exchanged per light atom and hyperfine pumping or mechanical effects can probe be neglected. The probe beam, whose diameter is typi- laser cally 1-2 mm, passes through the center of the spherical, roughly Gaussian-shaped atomic cloud. We then collect the diffuse light transmitted by the sample in a solid an- MOT gle 0.1 srd, as a function of the time elapsed after the probe is switched off. The diffuse light is detected by a SCP (Single Channel Plate). By adjusting the frequency of the molasses beams, we can tune the temperature of FIG. 1: Experimental setup. A cloud of laser cooled atoms the cloud i.e., the width of the atomic velocity distribu- released from a magneto-optical trap (MOT) is illuminated tion. Using this method, we can vary the ratio of the by a rectangular pulse of near resonant light. Part of the Doppler width to the homogenous (natural) width from transmitted diffuse light is detected as a function of time by 5 × 10−2 to 1. Attempts to further heat the gas re- a single channel plate (SCP). sulted in a drastic reduction of the steady-state atomic population in the MOT. The total number N of atoms in the cloud can be adjusted independently of temperature of quasi-coherent transport, corresponding to our lowest by switching off the repumper laser shortly before the temperature of 80µK. The comparison between resonant trapping beams. The time-resolved fluorescence signal and detuned excitation allows us to emphasize some pe- is averaged over 512 experimental cycles. The result- culiarities of RT in ultracold vapors. We then focus in ing signal-to-noise ratio allows us to observe the RT de- section IV on the role of Doppler frequency redistribution −2 cay up to approximately 10 of the initial, steady-state in our experimental situation. value of the diffuse transmission. We compare our ex- perimental data to a Monte-Carlo simulation which was II. DESCRIPTION OF EXPERIMENT AND initially developed to explain our coherent backscatter- SIMULATION ing signals [16]. The features of this numerical simulation are detailed in [17]. The new ingredient is the atomic ve- locity. For all atoms, we assume independent isotropic Gaussian velocity distributions, identical at all spatial In this section, we first briefly recall the main features positions. During propagation of a photon in the atomic of our experiment which was described in more details medium, the probability of the photon being scattered elsewhere [12]. We also shortly discuss the Monte-Carlo by an atom is chosen according the local atomic density simulation used to analyze our results. Radiation trap- (not uniform in the Gaussian-shaped atomic cloud), the ping experiments in atomic gases are immensely sim- atomic velocity distribution and the frequency of the in- plified by the fact that the time step for the random coming photon. The atomic velocity along the incoming walk of photons inside such media, the so-called trans- wave vector and the scattering probability are of course port time [14] in the regime of coherent transport, is of correlated: a photon with frequency red-detuned from the order of the lifetime of the excited state (typically a resonance is more likely to be scattered by an atom with few tens of nanoseconds). Even for samples of relatively velocity opposite to the wave vector of the photon, so low opacity, trapping times in excess of a microsecond that the frequency in the atomic rest frame is closer to are obtained. The requirements for pulsed excitation and resonance. This in turn introduces a correlation between time-resolved detection are thus much less stringent than the frequency of the incoming photon and the frequency in the case of non or weakly resonant samples [15]. of the scattered photon. At very low velocity, the fre- quency change at each scattering event is very small – The results presented in this paper are obtained us- much smaller than the natural linewidth Γ – making the ing the experimental configuration shown in Fig. 1. A photon frequency slowly changing along a multiple scat- cloudofupto1010 Rb85 atoms is collected in a magneto- tering path. In contrast, if the atomic velocity is large optical trap (MOT), then released and further cooled 2 enough – roughly when the Doppler shift kvrms is compa- down to T = mvrms/(3kB)=80µK using optical mo- rms rable or larger than Γ – the frequency shift at each scat- lasses.
Details
-
File Typepdf
-
Upload Time-
-
Content LanguagesEnglish
-
Upload UserAnonymous/Not logged-in
-
File Pages10 Page
-
File Size-