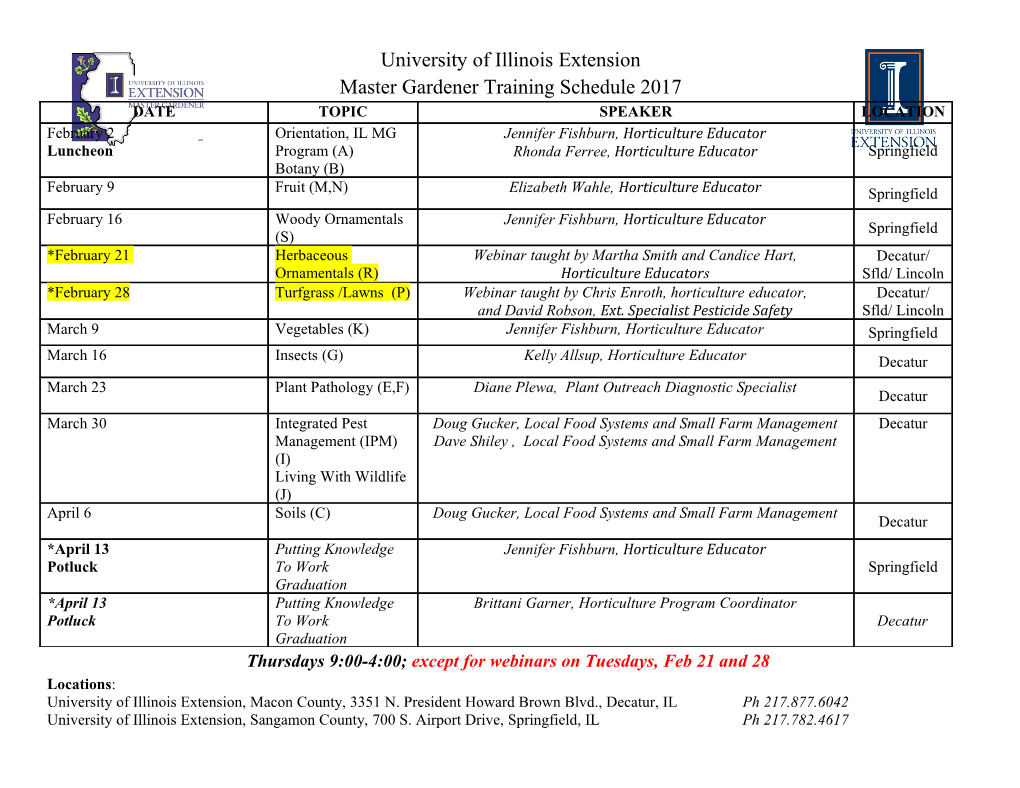
Phosphorylation-dependent structural changes in the N-terminal extension of SUR2A NBD1 By Clarissa Rana Sooklal A thesis submitted in conformity with the requirements for the degree of the Master of Science Graduate Department of Chemistry University of Toronto © Copyright by Clarissa Rana Sooklal, 2015 0 Phosphorylation-dependent structural changes in the N-terminal extension of SUR2A NBD1 Master of Science 2015 Clarissa Rana Sooklal Graduate Department of Chemistry University of Toronto Abstract ATP-sensitive potassium (KATP) channels are involved in many biological processes and play an important role in sustaining healthy functioning organs. KATP channels are composed of four copies of a pore-forming Kir6.x protein that is surrounded by four copies of regulatory sulfonylurea receptor (SUR) proteins. SUR proteins are members of the ATP-binding cassette (ABC) superfamily. Nucleotide binding and hydrolysis at the SUR proteins results in channel opening, which is potentiated by SUR protein phosphorylation. The studies conducted during this thesis aimed to understand how phosphorylation of the N-terminal extension (N-tail) of the first nucleotide binding domain (NBD1) of SUR2A alters its structure and influences the N-tail’s interaction with the core NBD1. This biophysical study utilizes nuclear magnetic resonance (NMR) and other biophysical techniques to understand these structural and dynamic changes in N-tail with phosphorylation which will allow us to further understand ATP-binding at NBD1 and control of KATP channel conductance. ii Acknowledgements I would first like to thank to my supervisor, Professor Voula Kanelis for all her support, guidance and direction. She has been my biggest inspiration and my role model throughout my graduate studies. Without her help and encouragement I would not have been able to expand my understanding of science and science research. Voula has been my teacher and mentor. And for that, I am forever grateful. I would also like to thank my past colleague Dr. Jorge Pédro Lopez-Alonso who first helped me get started with my project and find my way around the laboratory. Jorge not only made my transition into research an easy one, but also enjoyable. I would like to thank my current lab mates with an extra special thanks to Dr. Elvin de Araujo. His support and endless advice and help has been crucial in getting me through this project. Elvin was the greatest support and was willing to help whenever I ran into issues related to all aspects of my project from purifying the N-tail to using size-exclusion column chromatography and troubleshooting the NMR. And to Sasha Weiditch, who provided her support and comfort making the difficult times bearable. Throughout my graduate studies Sasha became my dear friend and her emotional support helped pull me through the stressful aspects of research. And I am truly grateful to Marijana, our lab technician for helping me obtain my N- tail’s DNA and her countless of favours. I would also like to thank Professor Jumi Shin for allowing me to use her circular dichroism instrument and equipment, and for reading my thesis. I would like to thank Professor Scott Prosser for allowing me to use his NMR magnet. And I would like to thank Dr. Darcy Burns and Dr. Sergiy Nokhrin for the help in conducting my 3D triples resonance NMR experiments and Dr. Peter Mitrakos for his help using a the HPLC at the University of Toronto Mississauga when I was troubleshooting the N-tail phosphorylation reaction experiments. And lastly, I would like to thank Richard Ramsundar and Llana Sooklal for their incredible and endless support! iii Table of Contents 1. Introduction 1 1.1 Biological importance of ABC transporters 1 1.2 Structural architecture of ABC transporters 1 1.3 The ABCC subfamily of ABC proteins and the sulfonylurea receptors (SURs) 3 1.4 KATP channels 4 1.5 Intrinsically disordered proteins 5 1.6 Phosphorylation regulation of KATP channels 6 1.7 N-terminal extension (N-tail) of SUR2A 6 1.8 Biophysical techniques to study SUR2A N-tail 7 1.8.1 Nuclear magnetic resonance 7 1.8.2 Circular dichroism 11 1.8.3 Dynamic light scattering 13 1.9 Resonance assignments 15 2. Materials and Methods 19 2.1 Expression and purification of SUR2A N-tail 19 2.2 Phosphorylation of SUR2A N-tail 20 2.3 Protein concentration determination 20 2.4 NMR spectroscopy 21 2.5 Circular dichroism 21 2.6 Size exclusion chromatography 22 2.7 Dynamic light scattering 22 3. Results 23 3.1 Analysis of the primary sequence of N-tail 23 3.2 Expression and purification of SUR2A N-tail S615-E664 construct 23 3.3 Biophysical characterization and structural studies of SUR2A N-tail-S615 26 3.3.1 NMR studies of non-phosphorylated N-tail-S615 26 3.3.2 Phosphorylation of N-tail-S615 27 3.3.3 Structural changes associated with the phosphorylation of N-tail-S615 31 iv 3.3.4 Changes in the interaction between N-tail-S615 and NBD1 with 35 phosphorylation 3.4 Expression and purification of SUR2A N-tail-S608 and N-tail-Q600 37 3.5 Non-phosphorylated and phosphorylated N-tail-S608 NMR spectra and other 41 characterization 3.5.1 Changes in the interaction between N-tail-S608 and NBD1 with 45 phosphorylation 3.6 Resonance Assignment of non-phosphorylated N-tail-S615 48 3.6.1 Selective unlabeling approach to assist in resonance assignment 51 3.6.2 Analysis of assigned N-tail-S615 54 4. Discussion 59 5. References 62 6. Copyright Permissions 68 v List of Tables Table 1. Brief list of the 7 ABC subfamilies in humans and examples 3 Table 2. List of the most important nuclei found in biomolecules and their gyromagnetic ratio 8 vi List of Figures Figure 1. Schematic of an ABC protein subfamily C (ABCC) 2 Figure 2. Schematic top view of KATP channel 5 Figure 3. Energy separation of nuclear spins in the presence of an external magnetic 8 field Figure 4. Example of a 2-dimensional 15N-HSQC spectrum 10 Figure 5. CD spectra of protein secondary structures 12 Figure 6. Comparison of the hydrodynamic radius under difference salt concentrations 13 monitored by DLS Figure 7. Effect of surface projections on the hydrodynamic radius 13 Figure 8. Determining the hydrodynamic radius of non-spherical particles 14 Figure 9. 3-dimensional NMR experiments used to assign resonances 17 Figure 10. 3-dimensional NMR experiments aided by CCC-TOCSY 3D experiment 18 Figure 11. Agadir predication of helicity based on N-tail-S615 primary sequence 23 Figure 12. SDS gel of the purification of a 1 L cell growth of 6xHis-SUMO N-tail-S615 24 fusion protein Figure 13. Gel sample of the purified N-tail-S615 used for NMR 24 Figure 14. Overlay of NBD1 (615-933) and N-tail-S615 TROSY 15N-HSQCs 26 Figure 15. 15N-HSQC NMR spectrum of 15N N-tail-S615 run at (A) 12 ºC and (B) 30 ºC 27 Figure 16. Monitoring the phosphorylation reaction of N-tail-S615 28 Figure 17. Gel filtration clean-up of (A) phosphorylated N-tail-S615 and 30 (B) phosphorylated N-tail-S615 HSQC Figure 18. SDS gel shift comparing non-phosphorylated and phosphorylated N-tail-S615 30 Figure 19. Comparison of non-phosphorylated and phosphorylated N-tail-S615 15N- 31 HSQCs Figure 20. Comparison of (A) native and (B) denatured non-phosphorylated and 32 phosphorylated N-tail-S615 gel filtrations Figure 21. DLS of non-phosphorylated and phosphorylated N-tail-S615 hydrodynamic 33 radii Figure 22. CD spectra of (A) non-phosphorylated and (C) phosphorylated N-tail-S615 34 with increasing concentrations of trifluoroethanol (TFE) Figure 23. Interaction experiment overlay of non-phosphorylated N-tail-S615 with and 36 without NBD115N-HSQCs vii Figure 24. Interaction experiment overlay of phosphorylated N-tail-S615 with and 37 without NBD115N-HSQCs Figure 25 SDS gel of the purification of a 1 L cell growth of 6xHis-SUMO N-tail-S608 39 fusion protein Figure 26 SDS gel of the purification of a 1 L cell growth of 6xHis-SUMO N-tail-Q600 40 fusion protein Figure 27. 15N-HSQC spectrum of 15N N-tail-S608 run at 12 ºC 41 Figure 28. 15N-HSQC spectrum of 15N N-tail-Q600 run at 12 ºC 41 Figure 29. Overlay of 3 non-phosphorylated N-tail constructs’ 15N-HSQC spectra 42 Figure 30. Overlay of non-phosphorylated and phosphorylated of N-tail-S608 15N- 43 HSQCs Figure 31. Overlay of non-phosphorylated and phosphorylated of N-tail-Q600 15N- 43 HSQCs Figure 32. Comparison of native non-phosphorylated and phosphorylated N-tail-S608 gel 44 filtrations Figure 33. CD spectra of (A) non-phosphorylated and (B) phosphorylated N-tail-S608 45 with increasing concentrations of TFE Figure 34. Interaction experiment overlay of non-phosphorylated N-tail-S608 with and 46 without NBD115N-HSQCs Figure 35. Interaction experiment overlay of phosphorylated N-tail-S608 with and 47 without NBD1 15N-HSQCs Figure 36. 3D NMR data used to link clusters and assign N-tail-S615 15N-HSQC peaks 49 Figure 37. Labeled resonance assignment on N-tail-S615 15N-HSQC 50 Figure 38. Overlay of selectively unlabeled lysine N-tail-S615 15N-HSQC 52 Figure 39. Reaction between iodoacetamide and peptide cysteine residue 53 Figure 40. N-tail-S615 modification with iodoacetamide 15N-HSQC 54 Figure 41. CSI calculation based on assigned chemical shift of N-tail-S615 55 Figure 42. Overlay of assigned non-phosphorylated N-tail-S615 and unassigned 55 phosphorylated N-tail 15N-HSQCs Figure 43. Interaction experiments analysis based on chemical shift, volume and intensity 57 changes vs.
Details
-
File Typepdf
-
Upload Time-
-
Content LanguagesEnglish
-
Upload UserAnonymous/Not logged-in
-
File Pages78 Page
-
File Size-