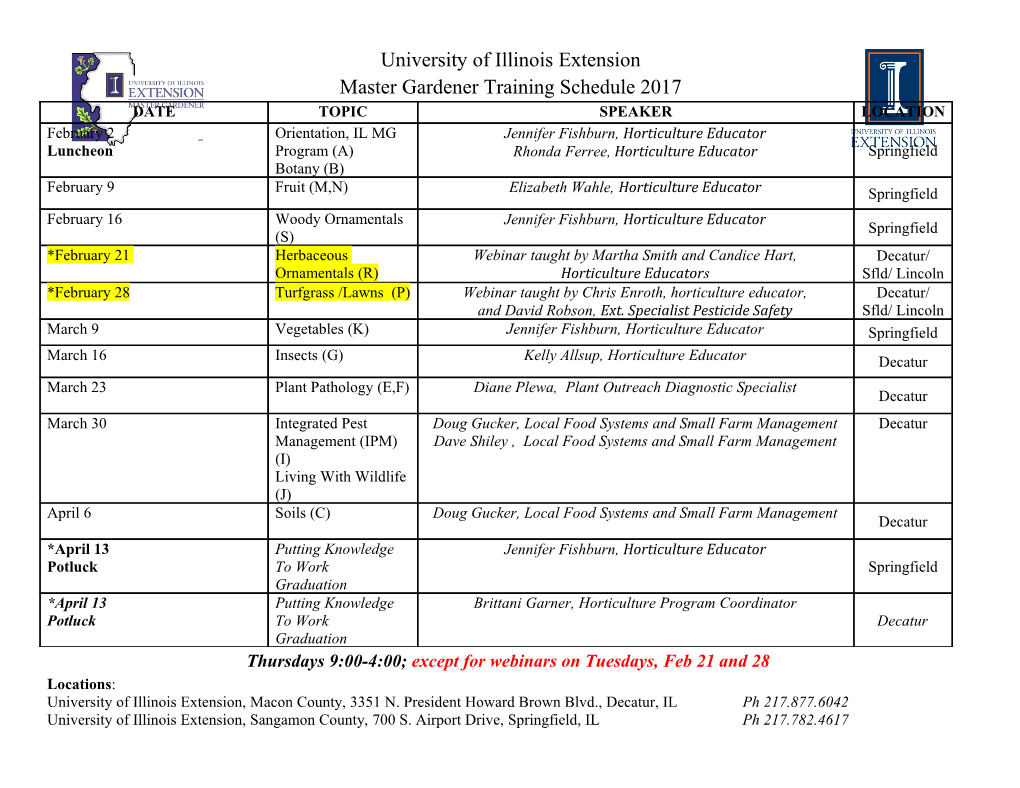
REFERENCE FRAMES AND EQUATIONS OF MOTION IN THE FIRST PPN APPROXIMATION OF SCALAR-TENSOR THEORIES OF GRAVITY A Dissertation presented to the Faculty of the Graduate School University of Missouri-Columbia In Partial Ful¯llment of the Requirements for the Degree of Doctor of Philosophy by IGOR VLASOV Dr. Sergei Kopeikin, Dissertation Supervisor MAY 2006 ACKNOWLEDGMENTS I am very grateful to my advisor Dr. Sergei Kopeikin for his endless energy, patience and positive attitude. Without his continuous encouragement and consultations this work would have never been completed. I am thankful to the faculty and sta® of the Department of Physics and Astronomy for a very worm and friendly atmosphere. I am also indebted to my family and friends whose love, care and support are always with me. ii Contents ACKNOWLEDGEMENTS ii LIST OF TABLES vi LIST OF ILLUSTRATIONS vii Chapter 1 Notations 1 2 Introduction 13 2.1 General Outline of the Dissertation . 13 2.2 Motivations and Historical Background . 14 3 Statement of the Problem 21 3.1 Field Equations in the Scalar-Tensor Theory of Gravity . 21 3.2 The Tensor of Energy-Momentum . 23 3.3 Basic Principles of the Post-Newtonian Approximations . 25 3.4 The Gauge Conditions and the Residual Gauge Freedom . 29 3.5 The Reduced Field Equations . 30 4 Global PPN Coordinate System 33 4.1 Dynamic and Kinematic Properties of the Global Coordinates . 33 4.2 The Metric Tensor and the Scalar Field in the Global Coordinates . 38 5 Multipolar Decomposition of the Metric Tensor and the Scalar Field in the Global Coordinates 41 5.1 General Description of Multipole Moments . 41 iii 5.2 Thorne-Blanchet-Damour Active Multipole Moments . 44 5.3 Thorne-Blanchet-Damour Scalar Multipole Moments . 47 5.4 Thorne-Blanchet-Damour Conformal Multipole Moments . 48 5.5 Post-Newtonian Conservation Laws . 50 6 Local PPN Coordinate System 53 6.1 Dynamic and Kinematic Properties of the Local Coordinates . 53 6.2 The Metric Tensor and the Scalar Field in the Local Coordinates . 56 6.2.1 The Scalar Field: Internal and External Solutions . 58 6.2.2 The Metric Tensor: Internal Solution . 58 6.2.3 The Metric Tensor: External Solution . 60 6.2.4 The Metric Tensor: The Coupling Terms . 66 6.3 Multipolar Decomposition of the Body's Gravitational Field in the Local Coordinates . 67 7 Parameterized Post-Newtonian Transformation from Local to Global Co- ordinates 73 7.1 Preliminary Remarks . 73 7.2 General Structure of the Coordinate Transformation . 76 7.3 Transformation of the Coordinate Bases . 79 8 Matching of the Post-Newtonian Expansions of the Metric Tensor and the Scalar Field 82 8.1 Historical Background . 82 8.2 Method of the Matched Asymptotic Expansions in the PPN Formalism . 85 8.3 Transformation of Gravitational Potentials from the Local to Global Coor- dinates . 88 8.3.1 Transformation of the Internal Potentials . 88 8.3.2 Transformation of the External Potentials . 93 8.4 Matching of the Scalar Field . 94 8.5 Matching of the Metric Tensor . 95 8.5.1 Matching of g00(t; x) andg ^®¯(u; w) in the Newtonian approximation 95 2 8.5.2 Matching of gij(t; x) andg ^®¯(u; w) to the order of O(² )...... 97 3 8.5.3 Matching of g0i(t; x) andg ^®¯(u; w) to the order of O(² )...... 100 4 8.5.4 Matching of g00(t; x) andg ^®¯(u; w) to the order of O(² )...... 103 iv 8.6 Final Form of the PPN Coordinate Transformation from Local to Global Coordinates . 108 9 Translational Equations of Motion of Extended Bodies 110 9.1 Introduction . 110 9.2 Macroscopic post-Newtonian Equations of Motion . 114 9.3 De¯nitions of Mass, the Center of Mass and the Linear Momentum of an Extended Body in the N-body System . 115 9.4 Translational Equation of Motion in Local Coordinates . 120 9.5 Equation of Translational Motion in Global Coordinates . 126 10 Rotational Equations of Motion of Extended Bodies 129 10.1 Post-Newtonian De¯nition of the Angular Momentum of the Body . 129 10.2 Equations of Rotational Motion in Local Coordinates . 130 11 Motion of Spherically-Symmetric and Rigidly Rotating Bodies 134 11.1 De¯nition of a Spherically-Symmetric and Rigidly Rotating Body . 134 11.2 Coordinate Transformation of Multipole Moments . 138 11.3 Multipolar Decomposition of Gravitational Potentials in Global Coordinates 140 11.4 Translational Equations of Motion . 141 11.5 Rotational Equations of Motion . 150 12 Conclusion 152 A Solution of the Laplace Equation for Scalar, Vector and Tensor Fields 153 B The Christo®el Symbols and the Riemann Tensor 157 B.1 The Christo®el Symbols . 157 B.2 The Riemann Tensor of External Gravitational Field in Local Coordinates 160 C Comparison with the Klioner-So®el Approach to Reference Frames in the PPN Formalism 161 BIBLIOGRAPHY 165 VITA 172 v List of Tables Table page 1.1 Particular Conventions and Symbols . 2 vi List of Figures Figure page 4.1 Astronomical N-body System . 34 4.2 Hierarchy of Coordinate Frames . 35 6.1 Two Hypersurfaces of Constant Time . 71 8.1 Lie transfer . 89 8.2 Two World Lines . 98 vii Chapter 1 Notations Greek indices ®; ¯; γ; ::: run from 0 to 3 and mark space-time components of four-dimensional objects. Roman indices i; j; k; ::: run from 1 to 3 and denote space components of three- dimensional objects. Repeated indices mean the Einstein summation rule, for instance, ® 0 1 2 3 k 1 2 3 A B® = A B0 + A B1 + A B2 + A B3, and Tk = T 1 + T 2 + T 3, etc. Minkowski metric is denoted ´®¯ = diag(¡1; +1; +1; +1). Kronecker symbol (the unit matrix) is denoted ±ij = diag(1; 1; 1). Levi-Civita fully antisymmetric symbol is "ijk such that "123 = +1. Kronecker symbol is used to raise and lower Roman indices. Complete metric tensor g®¯ is used to raise and lower the Greek indices in exact tensor equations whereas the Minkowski metric ´®¯ is employed for raising and lowering indices in the post- Newtonian approximations Parentheses surrounding a group of Roman indices mean symmetrization, for example, A(ij) = (1=2)(Aij + Aji). Brackets around two Roman indices denote antisymmetrization, that is A[ij] = (1=2)(Aij ¡ Aji). Angle brackets surrounding a group of Roman indices denote the symmetric trace-free (STF) part of the corresponding three-dimensional object, for instance, 1 1 1 1 A = A ¡ ± A ;A = A ¡ ± A ¡ ± A ¡ ± A : <ij> (ij) 3 ij kk <ijk> (ijk) 5 ij kpp 5 jk ipp 5 ik jpp We also use multi-index notations, for example, AL = Ai1i2:::il ;BP ¡1 = Bi1i2:::ip¡1 ;D<L> = D<i1i2:::il> : 1 Sum over multi-indices is understood as L i1i2:::il bL¡1 bi1i2:::il¡1 ALQ = Ai1i2:::il Q ;PaL¡1T = Pai1i2:::il¡1 T : ® ¡1 Comma denotes a partial derivative, for example, Á;® = @Á=@x , where Á;0 = c @Á=@t; Á;i = i ® @Á=@x ; and semicolon T ;¯ denotes a covariant derivative. L-order partial derivative with respect to spatial coordinates is denoted @L = @i1 :::@il . Other conventions are introduced as they appear in the text. We summarize these particular conventions and notations in the following table for the convenience of the readers: Table 1.1: Particular Conventions and Symbols Symbol Description Equation(s) g¹º physical (Jordan-Fierz frame) metric (3.1.4) tensor g~¹º conformal (Einstein frame) metric ten- (3.1.6) sor g determinant of g¹º (3.1.1) g~ determinant ofg ~¹º (3.4.2) ´¹º Minkowski (flat) metric tensor (3.3.4) ® ¡¹º Christo®el symbols (3.1.3) R¹º Ricci tensor (3.1.2) R Ricci scalar (3.1.1) 2 Symbol Description Equation(s) ~ R¹º conformal Ricci tensor (3.1.7) T¹º energy-momentum tensor of matter (3.1.2) T trace of the energy-momentum tensor (3.1.2) Á scalar ¯eld (3.1.1) Á0 background value of the scalar ¯eld Á (3.3.1) ³ dimensionless perturbation of the (3.3.1) scalar ¯eld θ(Á) coupling function of the scalar ¯eld (3.1.1) g Laplace-Beltrami operator (3.1.3) D'Alembert operator in the Minkowski (3.5.5) space-time ½ density of matter in the co-moving (3.2.1) frame ½¤ invariant (Fock) density of matter (3.3.18) ¦ internal energy of matter in the co- (3.2.1) moving frame ¼¹º tensor of (anisotropic) stresses of mat- (3.2.1) ter u® 4-velocity of matter (3.2.1) 3 Symbol Description Equation(s) vi 3-dimensional velocity of matter in the (3.3.15) global frame ! asymptotic value of the coupling func- (3.3.2) tion θ(Á) !0 asymptotic value of the derivative of (3.3.2) the coupling function θ(Á) c ultimate speed of general and special (3.1.1) theories of relativity ² small dimensional parameter, ² = 1=c (3.3.4) h¹º metric tensor perturbation, g¹º ¡ ´¹º (4.1.1) (n) n h ¹º metric tensor perturbation of order ² (3.3.4) in the post-Newtonian expansion of the metric tensor (2) N shorthand notation for h 00 (3.3.9) (4) L shorthand notation for h 00 (3.3.9) (1) Ni shorthand notation for h 0i (3.3.9) (3) Li shorthand notation for h 0i (3.3.9) (2) Hij shorthand notation for h ij (3.3.9) (2) H shorthand notation for h kk (3.3.9) 4 Symbol Description Equation(s) N;~ L~ shorthand notations for perturbations (5.4.1) of conformal metricg ~¹º γ "space-curvature" PPN parameter (3.5.1) ¯ "non-linearity" PPN parameter (3.5.2) ´ Nordtvedt parameter, ´ = 4¯ ¡ γ ¡ 3 (5.3.1) G observed value of the universal gravita- (3.5.4) tional constant G bare value of the universal gravitational (3.3.3), (3.5.4) constant x® = (x0; xi) global coordinates with x0 = ct and xi ´ x w® = (w0; wi) local coordinates with w0 = cu and wi ´ w U Newtonian gravitational potential in (4.2.1) the global frame U (A) Newtonian gravitational potential of (4.2.7) body A in the global frame Ui vector potential in the global frame (4.2.4) (A) Ui vector potential of body A in the global (4.2.7) frame 5 Symbol Description Equation(s) Â; ©1;:::; ©4 various special gravitational potentials (4.2.2),
Details
-
File Typepdf
-
Upload Time-
-
Content LanguagesEnglish
-
Upload UserAnonymous/Not logged-in
-
File Pages180 Page
-
File Size-