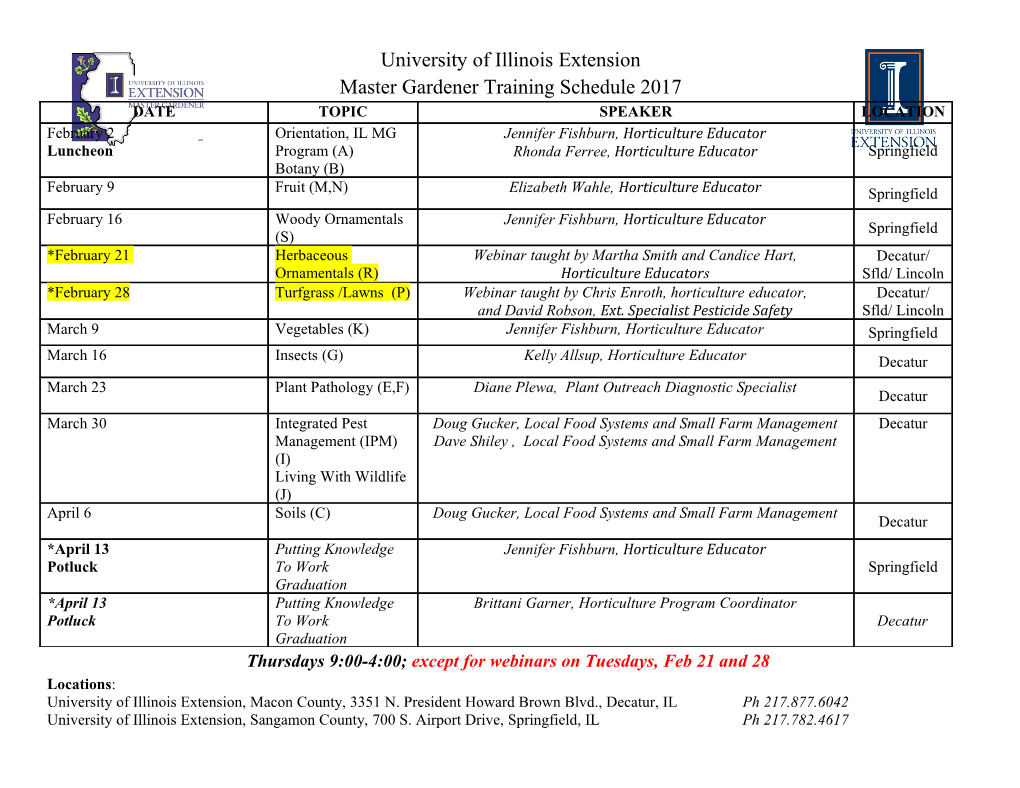
Manuscript Click here to view linked References 1 Middle-down tandem MS of glycopeptides 1 2 3 4 5 6 Comparison of collisional and electron-based dissociation modes for middle-down analysis of multiply 7 8 glycosylated peptides 9 1 2 3 1,2 #1 #1,3 10 Kshitij Khatri *, Yi Pu *, Joshua A. Klein , Catherine E. Costello , Cheng Lin , Joseph Zaia 11 12 13 14 1Dept. of Biochemistry, Center for Biomedical Mass Spectrometry, Boston University School of 15 Medicine 16 17 2 18 Dept. of Chemistry, Boston University 19 3 20 Program in Bioinformatics, Boston University 21 22 23 24 25 26 *Contributed equally 27 28 29 30 31 32 33 34 35 36 37 38 39 40 41 #Address for correspondence 42 43 44 Joseph Zaia/Cheng Lin 45 46 Boston University Medical Campus 47 48 670 Albany St., Rm. 509 49 50 Boston, MA 02118 51 52 (v) 1-617-638-6762 53 54 (f) 1-617-638-6761 55 56 57 (e) [email protected]; [email protected] 58 59 60 61 62 63 64 65 1 Middle-down tandem MS of glycopeptides 2 2 3 4 Abstract 5 6 Analysis of singly glycosylated peptides has evolved to a point where large-scale LC-MS analyses can be 7 8 9 performed at almost the same scale as proteomics experiments. While collisionally activated dissociation 10 11 (CAD) remains the mainstay of bottom-up analyses, it performs poorly for the middle-down analysis of 12 13 multiply glycosylated peptides. With improvements in instrumentation, electron-activated dissociation 14 15 (ExD) modes are becoming increasingly prevalent for proteomics experiments and for the analysis of 16 17 18 fragile modifications such as glycosylation. While these methods have been applied for glycopeptide 19 20 analysis in isolated studies, an organized effort to compare their efficiencies, particularly for analysis of 21 22 multiply glycosylated peptides (termed here middle-down glycoproteomics), has not been made. We 23 24 therefore compared the performance of different ExD modes for middle-down glycopeptide analyses. We 25 26 identified key features among the different dissociation modes and show that increased electron energy 27 28 29 and supplemental activation provide the most useful data for middle-down glycopeptide analysis. 30 31 32 Keywords: Tandem MS, glycoproteomics, middle-down, electron-activated dissociation, hotECD, 33 EThcD, FTICR-MS 34 35 36 37 38 39 40 41 42 43 44 45 46 47 48 49 50 51 52 53 54 55 56 57 58 59 60 61 62 63 64 65 1 Middle-down tandem MS of glycopeptides 3 2 3 4 Introduction 5 6 7 Electron-based activation of glycans and glycoconjugates yields informative product ions that 8 9 provide information critical to confident assignment of detailed structures and site-specific post- 10 11 12 translational modifications (1–5); however, the application of these methods to biological 13 14 samples has been limited by instrument availability, sensitivity and speed. Instrument and 15 16 workflow development needs to go hand-in-hand for efficient application and dissemination of 17 18 19 these methods. Here, we developed and tested approaches for standardization of sample 20 21 preparation and ExD (electron activated dissociation) analysis of multiply glycosylated peptides 22 23 24 (referred to here as middle-down glycopeptides) that are not characterized efficiently using 25 26 collisional dissociation methods. These efforts identified key analytical features for these 27 28 29 biopolymers using electron-based versus collisional dissociation modes. 30 31 Use of beam-type CAD (collisionally activated dissociation) for glycopeptide analysis requires 32 33 34 high collision energies to allow fragmentation of the peptide backbone. A particular problem 35 36 with such use of CAD is the low abundances of product ions from peptide backbone 37 38 fragmentation because the relatively fragile glycosidic bonds fragment easily. The glycan 39 40 41 composition can be inferred from the total mass of the precursor, once the peptide backbone has 42 43 been identified, but it may not always be possible to identify the exact site of modification if 44 45 46 peptide backbone fragments with attached saccharide units are not observed. This loss of the 47 48 fragile saccharide moiety poses a greater issue when analyzing multiply glycosylated peptides, 49 50 51 whereby the loss of saccharide from peptide backbone fragments prevents assignment of site- 52 53 specific glycan compositions. This limits the extent to which CAD-based methods can be applied 54 55 to analysis of heavily glycosylated peptides such as mucin-type O-glycosylated peptides, which 56 57 58 contain stretches of serine and threonine residues with multiple glycans attached, or peptides 59 60 61 62 63 64 65 1 Middle-down tandem MS of glycopeptides 4 2 3 4 with more than one N-glycosylation sequon. In some cases, it is possible to separate the multiple 5 6 7 sites of glycosylation using a combination of proteolytic enzymes; however, the usefulness of 8 9 CAD to analysis of multiply glycosylated peptides is limited. 10 11 12 We have previously developed analytical and bioinformatics workflows that combine 13 14 proteomics, glycomics and glycoproteomics information from bottom-up experiments to enable 15 16 efficient and comprehensive analysis of the glycoproteomes (6–8). When using a bottom-up 17 18 19 method, it is possible to determine the range of glycoforms present at each site but not to assign 20 21 the glycan combinations that exist simultaneously on different sites of the protein. In order to 22 23 24 determine the precise glycosylation structures of the functional proteoforms(s) of a glycoprotein, 25 26 it is necessary to develop methods for analysis of large and multiply glycosylated peptides; 27 28 29 however, this is challenging because the addition of glycans to proteins multiplies the total 30 31 number of molecular forms many-fold due to inherent glycosylation heterogeneity. In addition, 32 33 34 the number of protein-glycan combinations multiplies with an increase in the number of 35 36 glycosylation sites. Thus, for large glycoproteins with multiple glycosylation sites, only analysis 37 38 of peptides with 2-3 glycosylation sites and masses in the range 8,000 to 12,000 Da (defined here 39 40 41 as middle-down analysis) is feasible (6–10). 42 43 The challenge to analysis of multiply glycosylated peptides increases with size and number of 44 45 46 glycosylation sites. Methods for middle-down glycoprotein analysis are not yet mature and a 47 48 detailed comparison of ExD-based methods (9, 14, 14–23) combined with high-resolution and 49 50 51 high-sensitivity MS has not appeared. Multiply O-glycosylated synthetic peptides smaller than 7 52 53 kDa have been analyzed using ExD by direct infusion electrospray (2, 24). Generally, increased 54 55 m/z and number of glycosylation sites results in inefficient dissociation using ExD. The authors 56 57 58 show that this limitation can be overcome to a degree by increasing the electron energy in ECD 59 60 61 62 63 64 65 1 Middle-down tandem MS of glycopeptides 5 2 3 4 to achieve hECD (hot Electron Capture Dissociation). While their results indicate promise for 5 6 7 use of these methods for glycopeptide analysis, a direct comparison of dissociation techniques 8 9 for multiply glycosylated peptides with molecular weights exceeding 5-7 kDa is lacking. 10 11 12 The paucity of high-resolution and high-mass-accuracy ExD data on multiply glycosylated 13 14 peptides has also limited the development of efficient bioinformatics methodologies for 15 16 automated data analysis. In this work, we evaluated the performance of different fragmentation 17 18 19 modes for analysis of bottom-up and middle-down glycopeptides from glycoprotein standards 20 21 including human transferrin and human α1-acid glycoprotein (AGP). We made comparisons of 22 23 24 different dissociation modes systematically transitioning from bottom-up to middle-down 25 26 glycopeptide analyses, with particular focus on glycan heterogeneity, dissociation efficiency, 27 28 29 charge-state dependence and compatibility with online separation. The overall effort is geared at 30 31 identifying and applying the best methods for middle-down analysis that will help develop an 32 33 34 integrated workflow combining information from bottom-up and middle-down domains for the 35 36 most comprehensive glycoproteomic analysis. 37 38 39 Materials and methods 40 41 42 43 Sample preparation 44 45 For bottom-up glycopeptide analysis, human transferrin and human AGP (Sigma-Aldrich, St. 46 47 Louis, MO) were denatured by heating at 90oC for 30 min, in the presence of 2,2,2- 48 49 50 trifluoroethanol. Samples were reduced with dithiothreitol (DTT), alkylated using iodoacetamide 51 52 (IAM) and digested with Trypsin Gold (Promega Corp., Madison, WI) in the presence of 100 53 54 55 mM ammonium bicarbonate (Sigma-Aldrich, St. Louis, MO) as buffer. The detailed digestion 56 57 protocol has been described previously (6–8). Glycopeptides were enriched using a ZIC-HILIC 58 59 60 glycopeptide enrichment kit (EMD Millipore, Billerica, MA), as per the manufacturer’s protocol. 61 62 63 64 65 1 Middle-down tandem MS of glycopeptides 6 2 3 4 Samples were desalted, where necessary, using Pierce Pepclean C18 spin columns (Thermo 5 6 7 Fisher Scientific, Pittsburgh, PA). 8 9 For middle-down glycopeptide analysis, AGP was denatured, reduced, alkylated and digested 10 11 12 using Asp N endoproteinase (Promega Corp., Madison, WI). We evaluated both endoproteinase 13 14 Asp N and endoproteinase LysC for generation of middle-down glycopeptides from AGP. We 15 16 used Asp N for these studies because it showed superior reproducibility of digestion. Middle- 17 18 19 down glycopeptides were enriched by fractionation of the Asp N digest using a Superdex 75 20 21 (3.2/300) (GE Healthcare, Pittsburgh, PA) on a Beckman Gold HPLC system (Beckman Coulter, 22 23 24 Inc., Indianapolis, IN).
Details
-
File Typepdf
-
Upload Time-
-
Content LanguagesEnglish
-
Upload UserAnonymous/Not logged-in
-
File Pages32 Page
-
File Size-