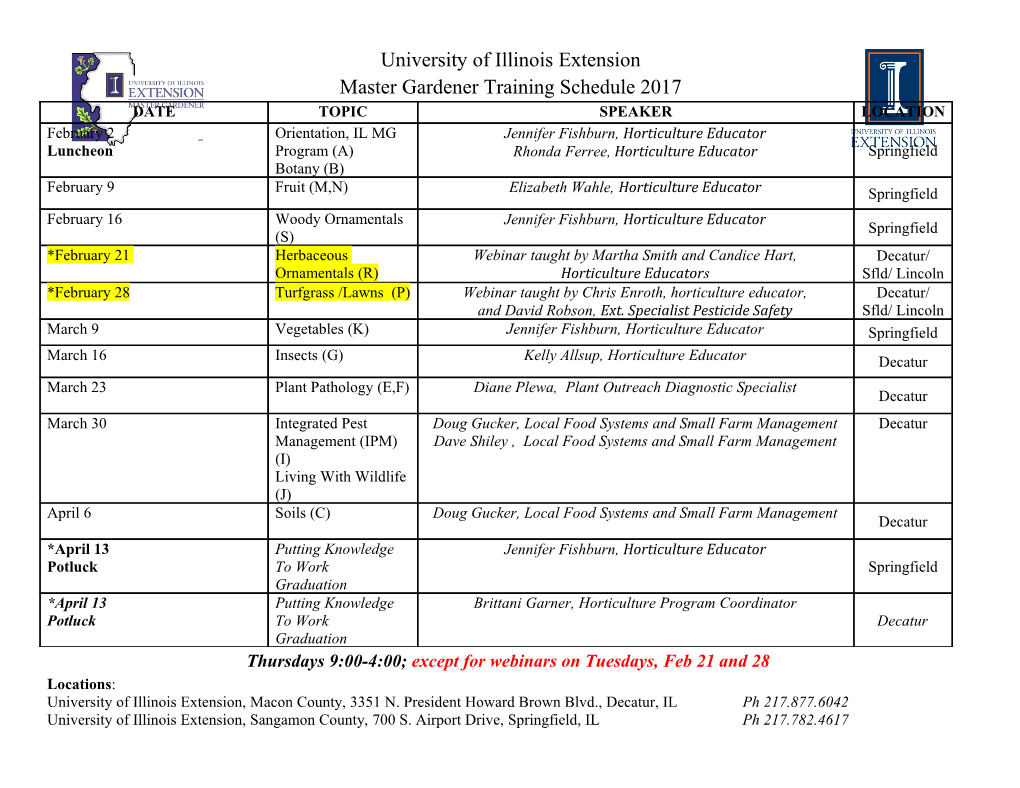
The Seismic Anisotropy of the Earth's Mantle: From Single Crystal to Polycrystal D. Mainprice, G. Barruol and W. Ben Ismaïl Laboratoire de Tectonophysique, CNRS, Université de Montpellier II, Montpellier, France. The anisotropic single crystal seismic properties are reviewed in the light of recent experimental and theoretical determinations. Although considerable progress has been made on the determination of single crystal properties, data are still lacking, particularly for the temperature derivatives of transition zone and lower mantle phases. The common types of LPO of olivine, opx and cpx are presented together with their associated seismic properties. It is emphasized that simple seismic symmetry pattern of upper mantle rocks are a direct result of the interaction of olivine, opx and cpx. Using the standard structural frame (X lineation, Z pole to foliation) for typical LPOs of olivine, opx and cpx have the maximum Vp parallel to X, in the XZ plane and parallel to Y respectively. Destructive interference occurs between these minerals and hence P-wave anisotropy should be sensitive to the aggregate composition. For shear wave splitting (dVs) typical olivine and opx LPOs result in similar patterns with the maximum dVs in the YZ plane and the fast split shear wave (Vs1) polarized parallel to the foliation. A typical cpx LPO on the other hand produces destructive interference as the max dVs is close to X. By comparison with experiments and numerical simulations, it is estimated that upper mantle samples have an olivine LPO strength which recorded shear strain gamma of between 0.25 and 2.0. Pyrolite and piclogite models are compared with global transverse isotropic models. The slowly reducing P-wave anisotropy in the first 200 km can be explained by a model with constant composition and LPO strength. The sharp decrease in the observed anisotropy in the global models cannot be explained by the transformation of opx to cpx at 300 km, it is proposed that this decrease is due to a reduction in LPO strength from 200 to 350 km at the base of the lithosphere. 1. INTRODUCTION Seismic anisotropy is a characteristic feature of the Earth, with anisotropy being present near the surface due to Earth's Deep Interior: Mineral Physics and Tomography aligned cracks (e.g. Crampin, 1984), in the lower crust, From the Atomic Scale to the Global Scale upper and lower mantle due to mineral preferred orientation Geophysical Monograph 117 (e.g. Mainprice and Nicolas, 1989, Karato, 1998). At the Copyright 2000 by the American Geophysical Union bottom of the lower mantle (D" layer, e.g. Kendall and Silver, 1998) and in the solid core (e.g. Morelli et al., 1986) 237 238 SEISMIC ANISOTROPY: FROM SINGLE CRYSTAL TO POLYCRYSTAL TEMPERATURE MINERALOGY DIRECT SAMPLES STRUCTURAL RADIAL SEISMIC & PRESSURE & PHASE CHANGES DIVISIONS ANISOTROPY VOLCANIC TEMPERATURE (°C) XENOLITHS 0 500 1000 1500 2000 OPHIOLITES 0.95 1.00 1.50 1.10 0 100 km 3 GPa 1100°C LITHOSPHERE SP6-F PREM ASTHENOSPHERE PYROXENES OLIVINE GARNETS DIAMOND V > V UPPER MANTLE SH SV 410 km 13 GPa 1400°C INCLUSIONS WADSLEYITE 500 MAJORITE TRANSITION ZONE V > V 660 km 23 GPa 1600°C RINGWOODITE SH SV ? V < V SH SV 1000 TEMPERATURE PRESSURE DEPTH (km) 1500 PEROVSKITES MAGNESIOWUSTITE LOWER MANTLE 2000 2500 2700 km 125 GPa 2000°C - 2500°C V > V 2900 km 135GPa D" LAYER SH SV 2500°C - 3500°C ? ? OUTER CORE NO S WAVES 3000 0 20 40 60 80 100 120 140 PRESSURE (GPa) Figure 1. The major physical and chemical variations in the Earth's mantle which control seismic anisotropy partly inspired after Gillet (1995). The seismic model SP6-F comes from Montagner and Kennett (1996). the causes of anisotropy are still controversial (Fig. 1). In stations, SKS studies show that the azimuth of the fast some cases, multiple physical factors could be contributing polarization direction is frequently parallel to the trend of to the measured anisotropy, for example mineral preferred mountain belts (see reviews from Silver, 1996 and Savage, orientation and alignment of melt inclusions at mid-ocean 1999). From the earliest observations, it was clear that the ridge systems (Mainprice, 1997). In the upper mantle, the anisotropy in the upper mantle was caused by the preferred pioneering work of Hess (1964) and Raitt et al. (1969) from orientation of olivine crystals induced by plastic deformation Pn velocity measurements in the shallow mantle of the related to mantle flow processes at the geodynamic or plate ocean basins showed azimuthal anisotropy. Long-period tectonic scale. surface waves studies (e.g. Nataf et al., 1984, Montagner and The major cause of seismic anisotropy in the upper Tanimoto 1990) have since confirmed that azimuthal and mantle is the crystal preferred orientation caused by plastic SH/SV polarization anisotropy are global phenomena in the deformation. Knowledge of the lattice preferred orientation Earth’s upper mantle, particularly in the top 200 km of the (L.P.O.) and its evolution requires well characterized sub-continental mantle. Anisotropic global tomography, naturally deformed samples, experimentally deformed based mainly on surface wave data, has shown that samples and numerical simulation for more complex anisotropy is very strong in the sub-continental mantle and deformation histories of geodynamic interest. The crystal present generally in the upper mantle but significantly preferred orientation not only causes seismic anisotropy, but weaker at greater depths. The large wavelengths used in long- also records some aspects of the deformation history. period surface waves studies means that such methods are Samples of the Earth's mantle are readily found on the insensitive to heterogeneity less than the wavelength of surface in the form of ultra-mafic massifs, xenoliths in about 1500 km. More recently in an effort to address the basaltic or kimberlitic volcanics and as inclusions in problem of regional variations of anisotropy the splitting of diamonds (Fig. 1). However, samples from depths greater SKS teleseismic shear waves which propagate vertically than 220 km are extremely rare. Upper mantle samples large have been extensively used (Kind et al., 1985, Silver and enough for the measurement of LPO have been recovered Chan 1988, 1991; Vinnik et al., 1989). At continental from kimberlitic volcanics in South Africa to a depth of MAINPRICE ET AL. 239 about 220 km established by geobarometry (e.g. Boyd, and to exsolution of Ca-perovskite from majorite garnet (Ita 1973). Kimberlite mantle xenoliths of deeper origin (>300 and Stixrude, 1992). The wadsleyite to ringwoodite km) with evidence for equilibrated majorite garnet which is transformation results in the lowering of anisotropy with now preserved as pyrope garnet with exsolved pyroxene have depth (see Table 1) in the lower part of the transition zone, been reported (Haggerty and Sautter, 1990; Sautter et al., whereas the exsolution of Ca-perovskite from majorite 1991). Samples of even deeper origin are preserved as garnet would result in an increase. Recent seismic studies by inclusions in diamonds. Although most diamonds crystallize Vinnik and co-workers (Vinnik and Montagner, 1996; at depths of 150-200 km, some diamonds contain inclusions Vinnik et al., 1997) show evidence for a weakly anisotropic of majorite (Moore and Gurney, 1985), enstatite and (1.5%) layer for S-waves at the bottom 40 km of the magnesiowüstite (Scott-Smith et al., 1984) and transition zone (620-660 km). The global tomography CaSiO3+(Fe,Mg)SiO3+SiO2 (Harte and Harris, 1993). The models (e.g. Montagner and Kennett, 1996; Montagner, mineral associations imply transition zone (410-660 km) and 1998) also show significant transverse isotropic anisotropy lower mantle origins for these diamond inclusions (Kesson in the transition zone with VSH > VSV (Fig. 1) and VPH > and Fitz Gerald, 1991). Although these samples help to VPV. Given the low intrinsic anisotropy of most of the constrain mantle petrology, they are too small to provide minerals in the lower part of the transition zone, Karato information about LPO. Hence knowledge of LPO in the (1998) suggested that this anisotropy is due to petrological transition zone and lower mantle will be derived from layering caused by garnet and ringwoodite rich layers of deformation experiments at high pressure and temperature transformed subducted oceanic crustal material. Such (e.g. Sharp et al., 1994; Dupas-Bruzek et al., 1998; Karato transversely isotropic medium with a vertical symmetry axis et al., 1998). would not cause any splitting for vertically propagating S- It has been accepted since the PREM seismic model waves. (Dziewonski and Anderson, 1981) that the top 200 km of the The strongest seismic discontinuity at 660 km is due to Earth's mantle is anisotropic on a global scale (Fig. 1). the dissociation of ringwoodite to perovskite and Indeed, a seismic discontinuity at about 200 km was first magnesiowüstite with a shear wave impedance contrast of reported by Lehmann (1959, 1961) which now bears her 9.9 % (e.g. Shearer 1996). The 660 km discontinuity has an name. However, the discontinuity is not always present at important topography with local depressions of up to 60 km the same depth. Anderson (1979) interpreted the from the global average in subduction zones (e.g. Flanagan discontinuity as the petrological change of garnet lherzolite and Shearer, 1998). From 660 to 1000 km a weak to eclogite. More recently, interpretations have favored an anisotropy is observed in the top of the lower mantle with anisotropy discontinuity, either a local anisotropic VSH < VSV (Fig. 1) and VPH < VPV (e.g. Montagner and decoupling shear zone marking the base of the lithosphere Kennett, 1996; Montagner, 1998). Karato (1998) attributed (Leven et al., 1981), a transition from an anisotropic mantle the anisotropy to the crystal preferred orientation of deforming by dislocation creep to isotropic mantle perovskite and possibly magnesiowüstite caused by plastic undergoing diffusion creep (Karato, 1992) or simply the base deformation in the convective boundary layer at the top of of an anisotropic layer beneath continents (Gaherty and the lower mantle.
Details
-
File Typepdf
-
Upload Time-
-
Content LanguagesEnglish
-
Upload UserAnonymous/Not logged-in
-
File Pages28 Page
-
File Size-