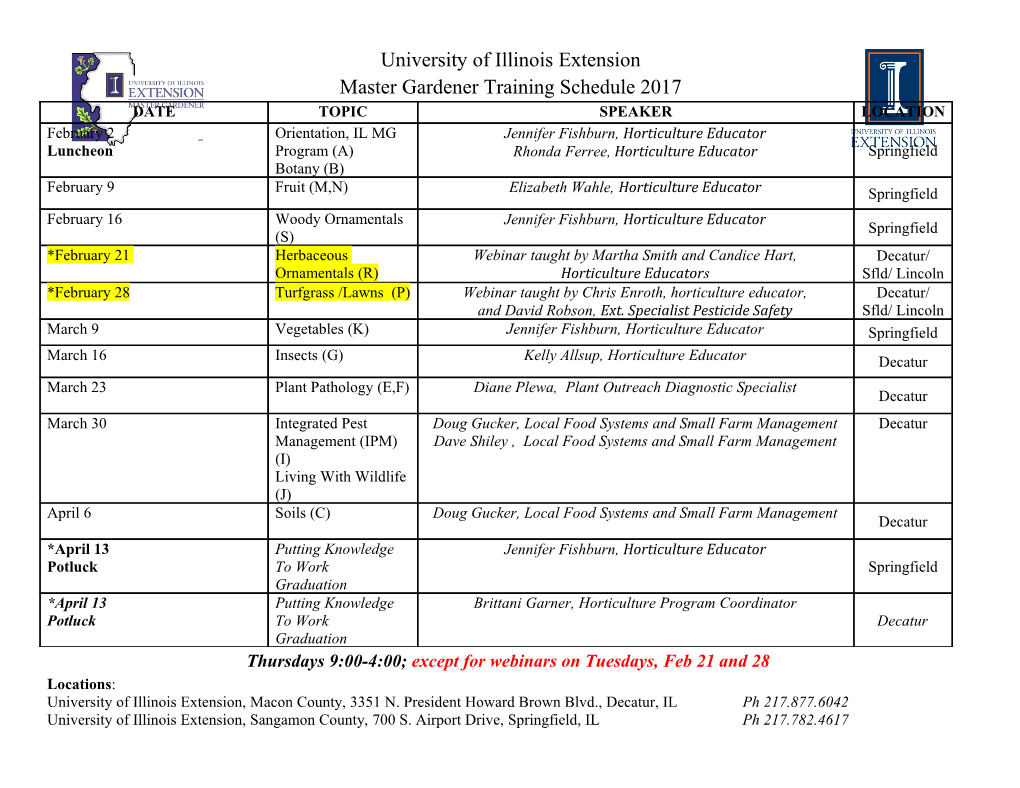
JULY 2013 Z H E N G E T A L . 1561 Influences of Sea Surface Temperature Gradients and Surface Roughness Changes on the Motion of Surface Oil: A Simple Idealized Study YANGXING ZHENG Center for Ocean–Atmospheric Prediction Studies, The Florida State University, Tallahassee, Florida MARK A. BOURASSA AND PAUL HUGHES Center for Ocean–Atmospheric Prediction Studies, and Department of Earth, Ocean and Atmospheric Science, The Florida State University, Tallahassee, Florida (Manuscript received 7 August 2012, in final form 7 March 2013) ABSTRACT The authors’ modeling shows that changes in sea surface temperature (SST) gradients and surface roughness between oil-free water and oil slicks influence the motion of the slick. Physically significant changes occur in surface wind speed, surface wind divergence, wind stress curl, and Ekman transport mostly because of SST gradients and changes in surface roughness between the water and the slick. These remarkable changes might affect the speed and direction of surface oil. For example, the strongest surface wind divergence (con- vergence) occurring in the transition zones owing to the presence of an oil slick will induce an atmospheric secondary circulation over the oil region, which in turn might affect the surface oil movement. SST-related changes to wind stress curl and Ekman transport in the transition zones appear to increase approximately linearly with the magnitude of SST gradients. Both surface roughness difference and SST gradients give rise to a net convergence of Ekman transport for oil cover. The SST gradient could play a more important role than surface roughness in changes of Ekman transport when SST gradients are large enough (e.g., several degrees per 10 km). The resulting changes in Ekman transport also induce the changes of surface oil movement. Sensitivity experiments show that appropriate selections of modeled parameters and geostrophic winds do not change the conclusions. The results from this idealized study indicate that the feedbacks from the surface oil presence to the oil motion itself are not trivial and should be further investigated for consideration in future oil-tracking modeling systems. 1. Introduction models is one method of tracking the spill. Many nu- merical ocean circulation models from different in- On 20 April 2010, the Deepwater Horizon oil plat- stitutions were used for trajectory forecasting [e.g., the form in the Gulf of Mexico suffered a catastrophic ex- West Florida Shelf model (Barth et al. 2008), the Global plosion that caused 11 deaths (Welch and Joyner 2010). Hybrid Coordinate Ocean Model (Global HYCOM; This explosion created a leak in the Macondo oil well Chassignet et al. 2007), the Gulf of Mexico HYCOM located approximately 1500 m below the sea surface, (http://www.hycom.org), the South Atlantic Bight–Gulf and the resulting oil spill presented an unprecedented of Mexico Model (SABGOM; Hyun and He 2010), the threat to Gulf of Mexico marine resources (Robertson Real-Time Ocean Forecast System for the North Atlantic and Krauss 2010). Tracking the oil spill both at the Ocean (RTOFS; Mehra and Rivin 2010), and the Intra- surface and at depth was necessary for planning miti- Americas Sea Nowcast/Forecast System (IASNFS; Ko gation efforts and for helping coastal regions to prepare et al. 2008)]. These models have been improved for for oil beaching. Trajectory forecasts using numerical tracking oil by taking advantage of the development of modern numerical models. However, their limitations were also obvious (Liu et al. 2011). For example, none of Corresponding author address: Yangxing Zheng, Center for Ocean–Atmosphere Prediction Studies, The Florida State Univer- these models incorporated Stokes drift (Stokes 1880), sity, 2035 E. Paul Dirac Dr., Johnson Building, Tallahassee, FL 32310. which is a transport due to the motion of waves. Stokes E-mail: [email protected] drift might play an important role in the motion of an oil DOI: 10.1175/JAMC-D-12-0211.1 Ó 2013 American Meteorological Society 1562 JOURNAL OF APPLIED METEOROLOGY AND CLIMATOLOGY VOLUME 52 slick, particularly in the nearshore region (Henaff et al. 2012). These forecasting models also did not consider biological consumption of oil or the physical–chemical weathering processes (Liu et al. 2011). In this paper, surface forcing due to two small-scale processes is examined through a highly idealized simu- lation. One change in surface forcing occurs because of SST gradients, and the other is related to changes in sur- face roughness. Global and regional numerical weather prediction (NWP) models were found to underestimate the significant influence of SST on near-surface winds in regions of strong SST gradients, which is evident in Quick Scatterometer (QuikSCAT) wind fields on spatial scales shorter than 1000 km (Chelton et al. 2001, 2004, 2007; O’Neill et al. 2003, 2005; Xie 2004; Chelton 2005; Chelton and Wentz 2005; Maloney and Chelton 2006; Song et al. 2009). These studies indicated the important FIG. 1. Schematic structure of UWPBL model in this study. influences of strong SST fronts on the near-surface winds on small spatial scales. SST gradients can be strong in the transition zones between oil-free water and surface oil because oil absorbs more solar radiation because of the theory) to a logarithmic profile (surface-layer theory). different thermal properties between oil and seawater. The matching conditions at the patch height between A thick oil slick was observed to exhibit an infrared the Ekman layer and the surface layer yield simple signature up to several degrees Celsius warmer than the similarity relations between the surface stress and the surrounding water during the Deepwater Horizon oil geostrophic flow. Stratification, baroclinicity, and sec- spill event (Svejkovsky et al. 2012). Second, physical ondary flows can be taken into account. The so-called processes affecting oil motion owing to the surface direct model is implemented in the experiments. If the roughness discrepancy between water and oil are not geostrophic winds and the necessary input variables fully considered in the forecast models. The sharp SST on the surface (e.g., SST, air surface temperature, hu- gradient and changes in surface roughness owing to the midity) are given, the direct model solves for the surface presence of an oil slick may cause additional or in- wind profile and PBL characteristics. The schematic creased ocean–atmosphere boundary processes that can structure of the UWPBL model for this study is shown influence the motion of the oil slick itself (e.g., through in Fig. 1. the variation of Ekman transport). Numerical models For this study, new momentum roughness length pa- for tracking oil do not consider these effects of oil on air– rameterizations are embedded in the model to solve the sea boundary processes, which ultimately affect the oil surface water roughness and the surface oil roughness. motion. This study will provide a basic understanding of The roughness length parameterizations for water and how strong SST gradient and roughness changes be- oil used in this model are different. For surface water tween surface oil and surface water affect the motion of roughness, the parameterization was first used in a study surface oil, and thus will improve oil trajectory forecasts by Bourassa (2006), which is a modification of the in future modeling. Bourassa–Vincent–Wood flux model (Bourassa et al. 1999). For surface oil roughness, the roughness length in this parameterization is modified to take oil features 2. Model and methodology into account. In this study, two major oil features that are different from seawater are considered: different a. UWPBL model surface roughness and the thermal properties distin- This study uses the University of Washington plane- guishable between oil and seawater. The model con- tary boundary layer (UWPBL) model, version 4.0 siders contributions to surface roughness from three types (Patoux and Brown 2002; Patoux et al. 2003), to examine of surface features that include an aerodynamically how temperature gradient and roughness changes be- smooth surface (Nikuradse 1933; Kondo 1975), capillary tween oil and surface water influence the motion of sur- waves (Bourassa et al. 1999), and gravity waves (Smith face oil. The wind profile in the boundary layer is resolved et al. 1992). The roughness length parameterization by patching a modified Ekman spiral (Ekman-layer equation for water/oil is JULY 2013 Z H E N G E T A L . 1563 5 21 0:11n bs 2 For water, Ulim 1.0 m s , and for the Deepwater 5 b 1 « b 21 z0 s c Horizon oil-covered region, Ulim 5 7.0 m s .Thisvalue i ju j r j jj Á j *i w u* u* ei of Ulim for oil was crudely chosen based on scatterometer aju jju Á e j 2 0:5 1 b * * i observations of the surface roughness of the Deepwater g , g Horizon spill. The value of Ulim is not general and will vary greatly depending on the oil conditions. Further b b b where the s, c, and g terms are weights for the work could be done to better estimate the values of Ulim roughness length associated with an aerodynamically and «; however, that is beyond the scope of this study. smooth surface, capillary waves, and gravity waves, re- Here our goal is to show that these considerations are spectively; n is the molecular viscosity of air; and « worthy of further analysis for at least the application of represents the oil damping effects on capillary waves modeling oil spill trajectories. and short gravity waves and here is set to 0.25 when oil is b. Experiment design present for most cases. We have conducted sensitivity experiments regarding the choice of «, for which the The model domain is 278–308N, 908–878W in the Gulf results are discussed in section 3f.
Details
-
File Typepdf
-
Upload Time-
-
Content LanguagesEnglish
-
Upload UserAnonymous/Not logged-in
-
File Pages15 Page
-
File Size-