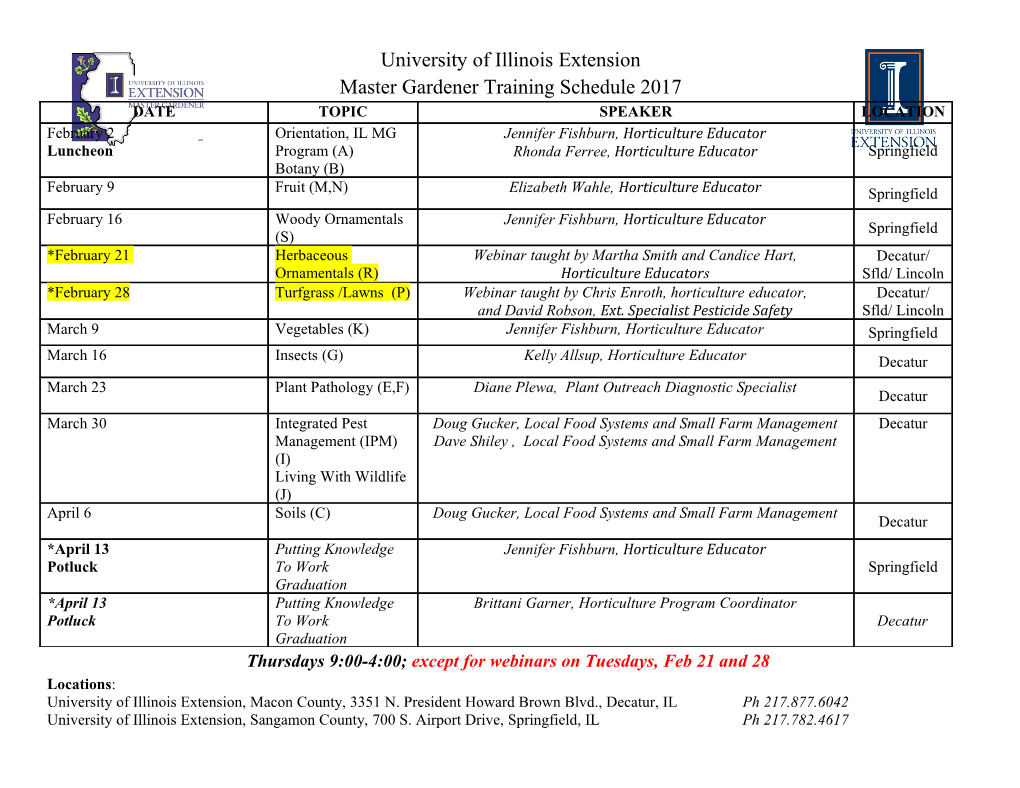
Modelling and optimisation of metal foam integrated heat exchangers for power electronics cooling Joseph Glass To cite this version: Joseph Glass. Modelling and optimisation of metal foam integrated heat exchangers for power elec- tronics cooling. Mechanical engineering [physics.class-ph]. Université Grenoble Alpes [2020-..], 2021. English. NNT : 2021GRALI007. tel-03203080 HAL Id: tel-03203080 https://tel.archives-ouvertes.fr/tel-03203080 Submitted on 20 Apr 2021 HAL is a multi-disciplinary open access L’archive ouverte pluridisciplinaire HAL, est archive for the deposit and dissemination of sci- destinée au dépôt et à la diffusion de documents entific research documents, whether they are pub- scientifiques de niveau recherche, publiés ou non, lished or not. The documents may come from émanant des établissements d’enseignement et de teaching and research institutions in France or recherche français ou étrangers, des laboratoires abroad, or from public or private research centers. publics ou privés. THÈSE Pour obtenir le grade de DOCTEUR DE L’UNIVERSITE GRENOBLE ALPES Spécialité : Mécanique des Fluides, Procèdes, Energétique Arrêté ministériel : 25 mai 2016 Présentée par Joseph GLASS Thèse dirigée par Didier BOUVARD, Professeur, Grenoble INP, et Codirigée par Yvan AVENAS, Maître de conférences, Grenoble INP, et Sébastien Ferrouillat, Maître de conférences, UGA Préparée au sein des Laboratoires SIMaP, LEGI, et G2Elab dans l'École Doctorale I-MEP2 Modelling and Optimisation of Metal Foam Integrated Heat Sinks for Power Electronics Cooling Thèse soutenue publiquement le 27 janvier 2021, devant le jury composé de : M. Frédéric TOPIN, Maître de conférences HdR, Aix-Marseille Université, Rapporteur M. Frédéric LEFEVRE Professeur, INSA Lyon, Rapporteur M. Remy DENDIEVEL Professeur, Grenoble INP, Président du Jury M. Paul-Etienne VIDAL Maître de conférences HdR, Ecole Nationale des Ingénieur de Tarbes, Examinateur M. Didier BOUVARD Professeur, Grenoble INP, Directeur de thèse M. Yvan AVENAS Maître de conférences HdR, Grenoble INP, Co-directeur de thèse, Invité M. Sébastien FERROUILLAT Maître de conférences, Université Grenoble-alpes, Co-encadrant, Invité ii ACKNOWLEDGEMENTS I would like to express my sincere gratitude to my supervisors Didier Bouvard, Yvan Avenas and Sébastien Ferrouillat for their invaluable support and guidance throughout the duration of my PhD. I would also like to thank Frédéric Girard, Florian Dumas and Sébastien Garcia for the large roles they played in the experimental aspects of this research project. I am also thankful for the support and encouragement I received from my friends and family during this period. Finally, I would like to thank my daughter Eloise, she is the reason for this undertaking, and is a constant motivation to me to do my best, to improve and to succeed. iii iv GENERAL INTRODUCTION Several decades ago, power electronics (PE) emerged as an important discipline in the world of electrical engineering. It became far more prevalent in industrial, commercial, residential, aerospace and military environments in a wide range of applications, such as heating control, AC and DC supplies, electrochemical processes, machine drives and harmonic filtering [1]. PE is described as the application of solid-state electronics to the control and conversion of electric power. In modern systems, this is carried out with semiconductor switching devices such as diodes, thyristors, and power transistors. In recent years, there has been revolution in the use of PE converters as wide band gap materials such as Silicon Carbide (SiC) and Gallium Nitride (GaN) have begun to replace the standard Silicon (Si) semiconductors. With these new materials, more compact and efficient devices can be fabricated that are capable of offering switching speeds of up to ten times faster than their counterparts. Improvements are also seen in the breakdown voltage, the operating temperature, the current and the switching loss over Si devices with the same die size and thickness [2]. However, though higher switching speeds can lead to improved power density and efficiency of the converter, these devices also suffer from electromagnetic interference (EMI) issues. One method of overcoming these problems was to fundamentally redesign the conventional packaging structure of the switching cell. Power Chip-on-Chip (PCoC) is a structure developed at G2Elab that superimposes the components of the switching cell, which greatly reduces parasitic inductances, and improves the overall electromagnetic compatibility (EMC) behaviour of the power module. Unfortunately, all gains in terms of EMC by the PCoC structure are paid for by a less efficient thermal management. This work thus aims to propose a solution to the problem of modern PE packaging by offering a compact and efficient cooling strategy, using metal foam as a novel heat sink within a forced-convection liquid cooling environment. The potential advantages to heat transfer of metal foams arise from the possibility of an increased specific surface area over other heat sinks, such as microchannels, and from the tortuous structure of metal foam that generates flow turbulence and improves convective transfers within the coolant. This work is a multidisciplinary endeavour carried out as a collaborated effort between G2Elab (power electronics, packaging and thermal management), LEGI (fluid mechanics, heat transfer, thermal and hydraulic modelling) and SIMaP (material engineering, sintering and microstructure characterisation) as part of the CoolPack project, funded by Univ. Grenoble Alpes. The resulting dissertation is organized as follows. Chapter 1 details the technological advancements in PE packaging and current cooling strategies, as well the characterisation and fabrication of metal foam. In Chapter 2, analytical models capable of predicting the thermal and hydraulic behaviour for metal foam and microchannel heat sinks are presented. In Chapter 3, the models are implemented into the software CADES to optimise the physical attributes of metal foam to maximises thermal performances whilst minimising the required hydraulic power. Finally, Chapter 4 contains a detailed look into the development of a new test bench and test section, capable of testing the thermal and hydraulic performances of small heat sinks. v vi CHAPTER I INTRODUCTION TO POWER ELECTRONICS PACKAGING AND THERMAL MANAGEMENT Table of Contents 1. INTRODUCTION ........................................................................................................................ 2 2. POWER ELECTRONICS PACKAGING .................................................................................. 2 3. OVERVIEW OF COOLING STRATEGIES ............................................................................. 7 3.1 NATURAL AND FORCED CONVECTION. .................................................................................. 8 3.2 HEAT PIPES ............................................................................................................................ 10 3.3 LIQUID COOLED COLD PLATES .............................................................................................. 10 3.4 TWO PHASE DIRECT COOLING ............................................................................................... 11 3.4.1 Spray cooling .................................................................................................................. 11 3.4.2 Jet impingement cooling ................................................................................................ 12 3.4.3 Immersion cooling .......................................................................................................... 12 3.5 INTEGRATED MICRO HEAT SINKS ......................................................................................... 13 3.5.1 Single- and two-phase microchannels ......................................................................... 13 3.5.2 Porous media cooling .................................................................................................... 14 4. SUMMARY OF COOLING STRATEGIES ........................................................................... 15 4.1 CHOICE OF THERMAL MANAGEMENT STRATEGY .................................................................. 15 4.2 PROJECT AIMS ........................................................................................................................ 16 5. METAL FOAMS ......................................................................................................................... 16 5.1 CLASSIFICATION .................................................................................................................... 17 5.2 MANUFACTURING TECHNIQUES........................................................................................... 18 5.2.1 Homogeneous pore distribution .................................................................................. 19 5.2.2 Non-homogenous pore distribution ............................................................................ 21 5.3 FOAM MATERIAL ................................................................................................................... 24 6. COOLANT ................................................................................................................................... 24 6.1.1 Types of Dielectric Coolant ........................................................................................... 25 6.1.2 Review of dielectric coolant thermal properties .......................................................
Details
-
File Typepdf
-
Upload Time-
-
Content LanguagesEnglish
-
Upload UserAnonymous/Not logged-in
-
File Pages150 Page
-
File Size-