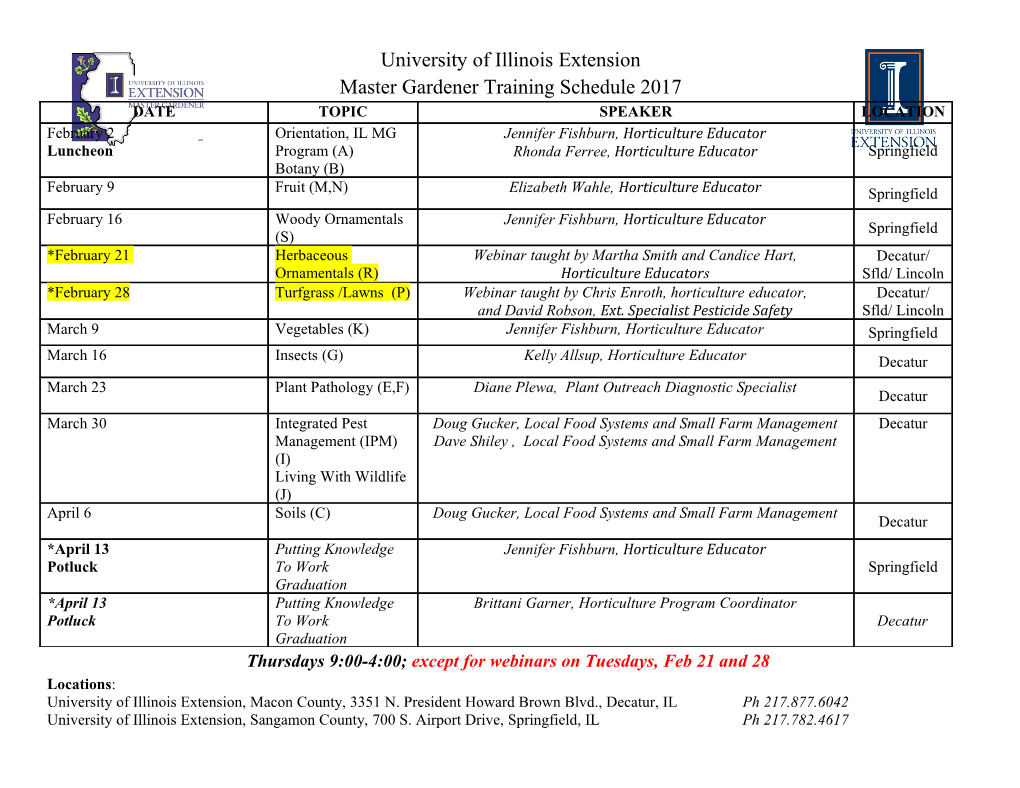
CRISM IMAGING SPECTROMETER Investigating Martian History with the CRISM Imaging Spectrometer Scott L. Murchie, Kevin J. Heffernan, Peter D. Bedini, David C. Humm, Patrick L. Thompson, Jeffrey Lees, Melissa J. Wirzburger, Hugo Darlington, John D. Boldt, Kim Strohbehn, David A. Lohr, John R. Hayes, Frank P. Seelos, Teck H. Choo, James A. McGovern, Erick Malaret, Christopher Hash, Peter J. Cavender, Debra L. Buczkowski, M. Frank Morgan, Kim D. Seelos, and Howard W. Taylor he Compact Reconnaissance Imaging Spectrometer for Mars (CRISM) is the pow- erful, technically innovative mineral-mapping camera on the Mars Reconnaissance Orbiter. The main objectives of the instrument are to map Mars’ crustal composi- tion and atmospheric processes, and to find and characterize past liquid water environ- ments that might have provided a habitat for life. CRISM was designed, built, and tested at APL and is currently being operated from the CRISM Science Operations Center at the Laboratory. In Martian orbit, CRISM is mapping the global distribution of Mars’ ices and minerals; measuring dust, ice, and trace gases in the atmosphere; and imaging several thousand key locations at high spatial and spectral resolution. First results are already changing our understanding of Martian history. TMARS, THE MOST EARTH-LIKE PLANET Ever since astronomers have peered at Mars through plateau known as Tharsis. Mars has tectonic features, telescopes, the red planet has fascinated scientists and fault zones and ridges formed by the movement of its the public for its potential similarity to Earth. From outer rigid layer, the lithosphere. The most conspicuous the beginning of spacecraft exploration of the planet tectonic features are long troughs surrounding Tharsis, with Mariner 4 in 1965, we have learned that Mars is including the mammoth Valles Marineris canyon system a transitional body, with characteristics between the whose length, breadth, and depth approximate the Med- highly evolved, active Earth and the geologically quiet iterranean if emptied of water. Like Earth, Mars rotates Moon.1 Mars’ surface consists of two main provinces: in about 24 h and is tilted on its axis, giving the planet old, densely cratered highlands that dominate the seasons. Each polar region has an ice cap consisting of southern hemisphere and younger, flat, low-lying plains alternating layers of cleaner and dustier water ice sitting that cover much of the northern hemisphere. Mars has atop stacked layers of sediments. The climate is extraor- the largest volcanoes in the solar system. Five of the dinarily cold and arid, with surface temperatures rarely biggest, with peaks 27 km above the northern plains, exceeding 0°C and dipping to –130°C during the polar are clustered on and around a North America–sized night. The average atmospheric pressure is only about JOHns HopKins APL TecHnical Digest, VolUme 27, NUMBer 2 (2006) 145 S. L. MURCHIE ET AL . 600 Pa (0.6% of Earth’s atmospheric pressure at sea level). The Mars Reconnaissance Orbiter (MRO)3 is the In most locations water cannot exist stably as liquid and seventh successful orbital mission to Mars. Its primary occurs instead only as ice or vapor. objectives are to Despite the thin, cold atmosphere, Mars resembles • Characterize present-day seasonal variations in water Earth in a way that is unique among other planets in our content of the surface and atmosphere solar system: many parts of its surface are carved by what • Search for evidence of past and present liquid water appear to be liquid water channels (Fig. 1). The chan- environments nels differ in their age and characteristics. Some date • Map the composition, geology, and layering of sur- to the planet’s earliest history about 4 billion years ago, face deposits whereas a few have formed within the last few years.2 3 The past occurrence of liquid water and its possible per- MRO has operated since November 2006 from a 255 sistence to the present, even under harsh surface condi- 320 km altitude, near-polar Sun-synchronous orbit that tions, provided an environment that may have met the crosses the equator at 3 p.m. local solar time. Its comple- conditions necessary for life. ment of instruments includes a high-resolution imager that can resolve surface features as small as a meter, a weather camera and an IR atmospheric sounder to mon- MRO AND CRISM itor clouds and trace gases, a radar to probe below the The effects of water on Mars’ surface are the cross-cut- surface for ice and water, and a mineral-mapping, vis- ting theme of NASA’s Mars Exploration Program. When ible-IR imaging spectrometer, the Compact Reconnais- 4 did water occur, and where does it still occur? How and sance Imaging Spectrometer for Mars (CRISM). for how long was the past climate different so as to permit In Martian orbit, CRISM is undertaking three major flowing water? How did water affect Mars’ geology, and did science investigations: it and does it provide a biological habitat? In the modern 1. Mapping the mineralogy of key areas with resolu- environment, how does water cycle between polar ice and tion as good as 15 m/pixel over the 362- to 3920-nm the atmosphere? To investigate these questions, a small wavelength range flotilla of NASA and European Space Agency (ESA) 2. Acquiring global imaging at a reduced spatial resolu- spacecraft have investigated Mars from the surface while tion of 200 m/pixel using 72 selected wavelengths to in orbit over the last decade (Fig. 2). provide contextual maps of key minerals Figure 1. (Left) 3.5–4.0-billion-year-old networks of river-cut valleys incise the Martian southern highlands. (Image courtesy of NASA/JPL- Caltech.) (Right) Gullies formed on crater and valley walls during the planet’s most recent history. (Image courtesy of NASA/JPL/Malin Space Science Systems.) 146 JOHns HopKins APL TecHnical Digest, VolUme 27, NUMBer 2 (2006) CRISM IMAGING SPECTROMETER Figure 2. Recent and planned Mars spacecraft. All were or will be launched by NASA, except for Mars Express, which was launched by ESA. 3. Measuring seasonal variations in dust, ice clouds, and objectives. One primary objective is high spatial resolu- trace gases in Mars’ atmosphere tion, better than 20 m/pixel. This is critical to seeing the layering of Mars’ rocks, which tell us the sequence CRISM consists of three assemblies (Fig. 3). The in which the rocks formed (younger rocks sit on top of largest, the optical sensor unit (OSU), contains optics, older rocks). CRISM must also take its images at high detectors, radiators, and a cryogenic system, all of which spectral resolution, in hundreds of wavelengths, to can be gimbaled. A data processing unit (DPU) provides determine which minerals make up the rocks. Electrons power, command and control, and data editing and in mineral crystals preferentially absorb light at specific compression, and gimbal motor electronics (GME) run energy levels, and the spectrum of reflected sunlight the gimbal. The OSU is the heart of CRISM. A 10-cm- exhibits absorption features at the corresponding wave- dia. Ritchey-Chretien telescope feeds a pair of Offner lengths. Numerous rock-forming minerals have distinc- convex-grating spectrometers. In each spectrometer, a tive signatures in reflectance spectra, and the minerals one-line spatial image is dispersed into the component present tell us about the environment in which the rocks wavelengths of reflected sunlight. One spectrometer, the formed (Fig. 4). For example, the iron- and magnesium- visible/near IR (VNIR), uses an array of silicon photodi- rich minerals olivine and pyroxene form in volcanic odes to capture its images. The other, an IR spectrome- ter, uses an array of HgCdTe diodes. Both detectors have a 640 3 480 (spatial 3 spectral) format of 27 mm2 pixels bonded to a TMC 6604A read-out integrated circuit. As MRO moves along its orbit, CRISM points to nadir to take images. To remove smear, the pivoting capability is used to track a point on the surface and scan across it slowly. A cryogenic cooling system maintains the IR detector near 2163°C for low noise, and the spec- trometer is cooled by a passive radiator to maintain low background. CRISM’s DESIGN AND FUNCTION CRISM’s design results from demanding engineer- ing requirements that must be met to fulfill its science Figure 3. Photo of the CRISM assemblies. JOHns HopKins APL TecHnical Digest, VolUme 27, NUMBer 2 (2006) 147 S. L. MURCHIE ET AL . in spacecraft attitude preclude passive cooling of the IR detector because a radiator would not have a consistent view to cold space. At the same time, a long-life pulse tube cooler would not fit into the instrument’s mass allocation. Meeting the instrument’s science requirements drove several technical innovations that solved these engi- neering challenges and allowed CRISM to double as a high-resolution imager and global mapper while main- taining the high quality of both data types.5 • When CRISM observes a target at high resolution, the gimbal actively tracks the target while simulta- neously scanning across it slowly. In this way longer exposure times can be used to build up a high SNR without smearing the image. • An internal integrating sphere (a sandblasted alumi- num sphere containing an internal lamp with a small Figure 4. Major mineral indicators of the environments in which aperture through which the illuminated interior is Martian rocks formed have distinctive spectral signatures at vis- viewed) provides a stable radiometric reference that ible to IR wavelengths. allows instrument artifacts to be calibrated out. • The DPU performs data editing and compression. CRISM takes its lower-resolution contextual map rock. Pyroxene persists in erosional debris, but olivine by parking the gimbal at nadir, spatially binning the quickly weathers when water is present, so its presence images, and returning only selected wavelengths.
Details
-
File Typepdf
-
Upload Time-
-
Content LanguagesEnglish
-
Upload UserAnonymous/Not logged-in
-
File Pages11 Page
-
File Size-