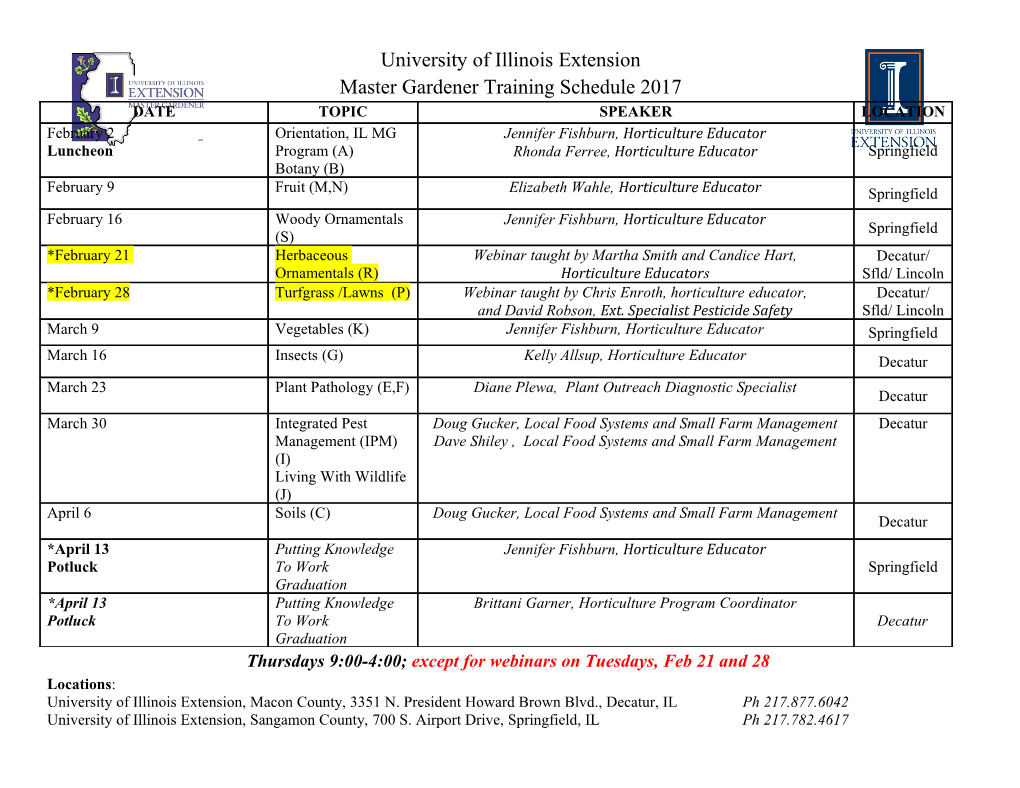
Increased photosystem II stability promotes H2 production in sulfur-deprived Chlamydomonas reinhardtii Alena Volgusheva1, Stenbjörn Styring, and Fikret Mamedov2 Molecular Biomimetics, Department of Chemistry-Ångström Laboratory, Uppsala University, 751 20 Uppsala, Sweden Edited by Harry B. Gray, California Institute of Technology, Pasadena, CA, and approved March 18, 2013 (received for review November 28, 2012) Photobiological H2 production is an attractive option for renewable creating anaerobic conditions in the cell. This in turn activates solar fuels. Sulfur-deprived cells of Chlamydomonas reinhardtii have hydrogenase expression and activity (10, 11). been shown to produce hydrogen with the highest efficiency Cells of C. reinhardtii undergo morphological changes and ac- among photobiological systems. We have investigated the photo- cumulate starch under S deprivation (12). Both the light and the synthetic reactions during sulfur deprivation and H2 production in dark reactions of photosynthesis are down-regulated (9, 12, 13). the wild-type and state transition mutant 6 (Stm6) mutant of Chla- The amount of Rubisco is drastically reduced during the first 24 h, mydomonas reinhardtii . The incubation period (130 h) was dissected leading to cessation of the CO2 fixation (12, 14). Photosynthetic into different phases, and changes in the amount and functional electron transfer reactions are also inhibited (9, 15, 16). This is status of photosystem II (PSII) were investigated in vivo by electron mostly associated with changes in the photosystem II (PSII) paramagnetic resonance spectroscopy and variable fluorescence complex that are not well characterized. This is unfortunate be- measurements. In the wild type it was found that the amount of cause a fraction of the electrons delivered to the hydrogenase have PSII is decreased to 25% of the original level; the electron transport been suggested to originate from water oxidation in PSII (17). from PSII was completely blocked during the anaerobic phase pre- PSII is a large multisubunit complex that drives photooxidation ceding H2 formation. This block was released during the H2 produc- of water and reduction of the plastoquinone (PQ) pool, thus ini- tion phase, indicating that the hydrogenase withdraws electrons tiating the electron transport chain in the thylakoid membrane BIOCHEMISTRY from the plastoquinone pool. This partly removes the block in PSII (18). Apart from light capture and charge separation, PSII pos- fl electron transport, thereby permitting electron ow from water sesses a defined sequence of electron and proton transfer reactions oxidation to hydrogenase. In the Stm6 mutant, which has higher (19). In addition, the PSII complex undergoes complex regulation respiration and H2 evolution than the wild type, PSII was analo- in response to environmental stress factors, especially high light gously but much less affected. The addition of the PSII inhibitor 3- intensities (20, 21). In this study we analyze the changes in the PSII (3,4-dichlorophenyl)-1,1-dimethylurea revealed that ∼80% of the content and activity under H production conditions in the wild- H production was inhibited in both strains. We conclude that (i) 2 2 type (WT) and the Stm6 mutant of C. reinhardtii. The latter was at least in the earlier stages, most of the electrons delivered to the earlier reported to exhibit enhanced H yield.* We report and hydrogenase originate from water oxidation by PSII, (ii) a faster 2 onset of anaerobiosis preserves PSII from irreversible photoinhibi- quantify the decrease in the amount of PSII and describe a regu- tion, and (iii) mutants with enhanced respiratory activity should be lation of the acceptor side reactions in PSII. The latter is con- considered for better photobiological H production. trolled by changes in the redox state of the quinone acceptors in 2 PSII and of the PQ pool occurring during S deprivation and sub- sequent H production. olar fuels are an attractive concept for development of future 2 Srenewable energy systems. Among other fuels, H2 is consid- Results ered to be one of the most effective and clean fuels (1–3). Solar- O2 and H2 Balance During S Deprivation of WT and Stm6 Mutant Cells. driven H2 production by photosynthetic microorganisms (photo- Under photoheterotrophic conditions, the growth rates of the WT bio-H2 production) is a viable alternative that complements the and the Stm6 mutant strains were not different. After the cells proposed chemical technologies. Green algae and cyanobacteria were transferred to the S-deprived conditions, the cell number can, using water as an electron source via photosynthesis, produce decreased by less than 10% by the end of the experiment. The fi H2 with speci cH2-evolving enzymes (hydrogenases) coupled to total amount of chlorophyll (Chl) also decreased by ∼20%, and the photosynthetic machinery (4–6). the Chl a/b ratio increased from 2.60 to 3.06 for the WT and from Although green algae possess a very active hydrogenase enzyme 2.30 to 2.94 for the Stm6 mutant. This is explained by partial compared with other organisms (the turnover rate of the algal Fe- degradation of the LHCII antenna (12). Fe hydrogenase is in thousands per second, 100-fold higher than In both the WT and the Stm6 mutant cells, the rate of O evo- fi 2 that of other hydrogenases), direct light-to-H2 conversion ef - lution significantly decreased and the rate of respiration increased ciency is very low (7, 8). Thus, this is not a main metabolic process. Moreover, H2 formation requires anaerobic conditions in the cell because the hydrogenase activity is sensitive to the presence of O2. Author contributions: S.S. and F.M. designed research; A.V. and F.M. performed research; The consequence is that oxygenic photosynthesis cannot easily be A.V., S.S., and F.M. analyzed data; and A.V., S.S., and F.M. wrote the paper. fl directly coupled to H2 evolution in green algae. The authors declare no con ict of interest. Melis and coworkers reported a two-stage process based on This article is a PNAS Direct Submission. sulfur (S) deprivation in Chlamydomonas reinhardtii, which 1Present address: Department of Biophysics, Faculty of Biology, Lomonosov Moscow State University, 119991 Moscow, Russia. allowed the separation of the photosynthetic reactions from H2 formation (9). Cell cultivation in sealed conditions in media de- 2To whom correspondence should be addressed. E-mail: fi[email protected]. fi prived of sulfur allowed light-dependent H2 evolution for several *The Stm6 mutant (state transition mutant 6) with modi ed light harvesting antenna days. The efficiency was the highest for photobiological systems properties and lacking cyclic electron flow was first isolated and described in (22). The mutant is lacking the Moc1 gene involved in the assembly of the mitochondrial respi- reported so far (2%) (8). Under these conditions photosynthesis is ratory chain in the light (22) and has been shown to have extended H2 production under down-regulated and O2 consumption overtakes O2 evolution, S deprivation (23). www.pnas.org/cgi/doi/10.1073/pnas.1220645110 PNAS Early Edition | 1of6 Downloaded by guest on September 25, 2021 Fig. 1. Changes in the concentration of dis- fi A C solved O2 ( lled circles) and produced H2 (open 600 circles) during incubation of WT (A, black circles) 12 III IV and the Stm6 mutant (B,redcircles)ofC. rein- M 100% 40 400 ], hardtii under S-deprived conditions in sealed 2 8 ], mM 1 /L of culture 2 2 flasks. Reflecting the O2 and H2 concentrations, [O 200 [H the time course was divided into four phases: I— 4 30 III the O evolution phase; II—the O consumption 2 2 mmol H 0 0 phase; III—the anaerobic phase; and IV—the H2 20 production phase. The blue stars in each phase B (mg Chl x h) III IV 2 fl 600 indicate the time points for EPR and uorescence 12 = experiments. The sample at t 0wastakenas M 100% 22% 10 mol H acontrol.(C) The effect of the addition of 20 μM 400 ], 8 ], mM /L of culture 2 2 DCMUontherateofH2 production. The aliquots 2 21% [O 200 [H of the cell culture were taken as described in the 4 0 text at the time points indicated by arrows in A I II WT Stm6 mmol H and B. The amount of H2 formed in the respective 0 0 strains in the absence of DCMU (blue) is set as 0 153045607590105120 S-deprivation, h 100%. The fraction of H2 formed in the presence of DCMU (red) was similar in both WT and the Stm6 mutant whereas the net amount of H2 formedwasfourtimesgreaterinthemutant. in the beginning of S deprivation, leading to establishment of an- in Fig. 1A (WT) and Fig. 1B (Stm6 mutant) and incubated in the aerobic conditions in the culture. Interestingly, due to the lower presence or absence of DCMU. In both the WT and the Stm6 ∼ initial rate of O2 evolution and the higher initial rate of respiration mutant, 80% of the rate of H2 evolution was lost upon the ad- in the Stm6 mutant, anaerobiosis was achieved much faster in the dition of DCMU (Fig. 1C). Thus, our results indicate a significant Stm6 mutant cells than in the WT cells. Fig. 1 A and B shows the involvement of PSII in photosynthetic hydrogen evolution. concentration of dissolved O2 in the medium during S deprivation The molecular mechanism of how this occurs has been addressed in the sealed flasks. First, the concentration of O2 in the medium here with selective spectroscopic analysis. Measuring points to as- increased due to still photosynthetically active cells during this sess PSII reactions were selected during the four different phases A B period. After this, the concentration of O2 started to decrease, (indicated by stars in Fig. 1 and ), and at each time point finally reaching anaerobicity.
Details
-
File Typepdf
-
Upload Time-
-
Content LanguagesEnglish
-
Upload UserAnonymous/Not logged-in
-
File Pages6 Page
-
File Size-