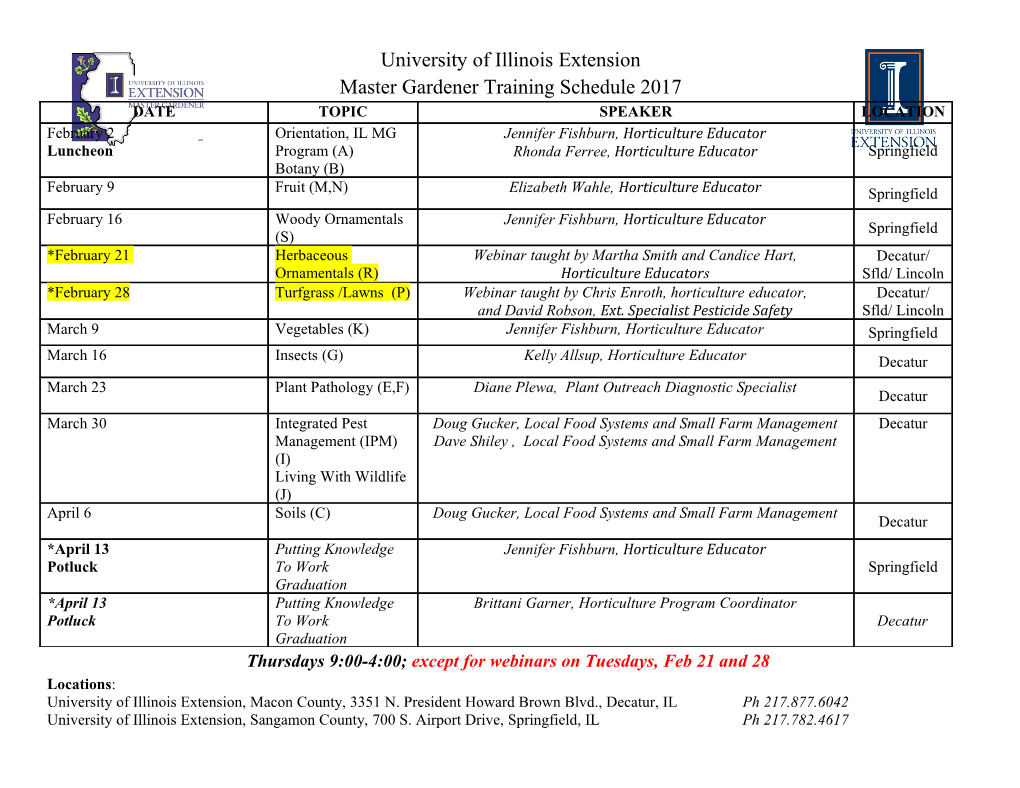
BMLT-2nd SEM Biochemistry & Biophysics: Paper-V, Unit-9 Topic: PROTEIN METABOLISM Submitted by: Mousumi Mitra 1 | P a g e PROTEIN METABOLISM Amino acid catabolism is part of the larger process of the metabolism of nitrogen-containing molecules. Nitrogen enters the body in a variety of compounds present in food, the most important being amino acids contained in dietary protein. Nitrogen leaves the body as urea, ammonia, and other products derived from amino acid metabolism. The role of body proteins in these transformations involves two important concepts: the amino acid pool and protein turnover. A. Amino acid pool Free amino acids are present throughout the body, for example, in cells, blood, and the extracellular fluids. For the purpose of this discussion, envision all these amino acids as if they belonged to a single entity, called the amino acid pool. This pool is supplied by three sources: 1) amino acids provided by the degradation of body proteins, 2) amino acids derived from dietary protein, and 3) synthesis of nonessential amino acids from simple intermediates of metabolism. Conversely, the amino pool is depleted by three routes: 1) synthesis of body protein, 2) amino acids consumed as precursors of essential nitrogen-containing small molecules, and 3) conversion of amino acids to glucose, glycogen, fatty acids, ketone bodies, or CO2 + H2O. Although the amino acid pool is small (comprised of about 90–100 g of amino acids) in comparison with the amount of protein in the body (about 12 kg in a 70-kg man), it is conceptually at the center of whole-body nitrogen metabolism. B. Protein turnover Most proteins in the body are constantly being synthesized and then degraded, permitting the removal of abnormal or unneeded proteins. For many proteins, regulation of synthesis determines the concentration of protein in the cell, with protein degradation assuming a minor role. For other proteins, the rate of synthesis is constitutive, that is, relatively constant, and cellular levels of the protein are controlled by selective degradation. DIGESTION OF DIETARY PROTEINS Most of the nitrogen in the diet is consumed in the form of protein, typically amounting to 70–100 g/day in the American diet. Proteins are generally too large to be absorbed by the intestine. They must, therefore, be hydrolyzed to yield di- and tripeptides as well as individual amino acids, which can be absorbed. Proteolytic enzymes responsible for degrading proteins are produced by three different organs: the stomach, the pancreas, and the small intestine. A. Digestion of proteins by gastric secretion The digestion of proteins begins in the stomach, which secretes gastric juice—a unique solution containing hydrochloric acid and the proenzyme, pepsinogen. 2 | P a g e 1. Hydrochloric acid: Stomach acid is too dilute (pH 2–3) to hydrolyze proteins. The acid, secreted by the parietal cells, functions instead to kill some bacteria and to denature proteins, thus making them more susceptible to subsequent hydrolysis by proteases . 2. Pepsin: This acid-stable endopeptidase is secreted by the chief cells of the stomach as an inactive zymogen (or proenzyme), pepsinogen. In general, zymogens contain extra amino acids in their sequences that prevent them from being catalytically active. [Note: Removal of these amino acids permits the proper folding required for an active enzyme.] Pepsinogen is activated to pepsin , either by HCl, or autocatalytically by other pepsin molecules that have already been activated. Pepsin releases peptides and a few free amino acids from dietary proteins. B. Digestion of proteins by pancreatic enzymes On entering the small intestine, large polypeptides produced in the stomach by the action of pepsin are further cleaved to oligopeptides and amino acids by a group of pancreatic proteases. 1. Specificity: Each of these enzymes has a different specificity for the amino acid R-groups adjacent to the susceptible peptide bond. For example, trypsin cleaves only when the carbonyl group of the peptide bond is contributed by arginine or lysine. These enzymes, like pepsin described above, are synthesized and secreted as inactive zymogens. 2. Release of zymogens: The release and activation of the pancreatic zymogens is mediated by the secretion of cholecystokinin and secretin, two polypeptide hormones of the digestive tract. 3. Activation of zymogens: Enteropeptidase (formerly called enterokinase ) —an enzyme synthesized by and present on the l uminal surface of intestinal mucosal cells of the brush border membrane—converts the pancreatic zymogen trypsinogen to trypsin by removal of a hexapeptide from the N-terminus of trypsinogen. Trypsin subsequently converts other trypsinogen molecules to trypsin by cleaving a limited number of specific peptide bonds in the zymogen. 4. Abnormalities in protein digestion: In individuals with a deficiency in pancreatic secretion (for example, due to chronic pancreatitis, cystic fibrosis, or surgical removal of the pancreas), the digestion and absorption of fat and protein are incomplete. This results in the abnormal appearance of lipids and undigested protein in the feces. C. Digestion of oligopeptides by enzymes of the small intestine The luminal surface of the intestine contains aminopeptidase— an exopeptidase that repeatedly cleaves the N-terminal residue from oligopeptides to produce even smaller peptides and free amino acids. 3 | P a g e D. Absorption of amino acids and small peptides Free amino acids are taken into the enterocytes by a Na+-linked secondary transport system of the apical membrane. Di- and tri- peptides, however, are taken up by a H+-linked transport system. The peptides are hydrolyzed in the cytosol to amino acids that are released into the portal system by facilitated diffusion. Thus, only free amino acids are found in the portal vein after a meal containing protein. These amino acids are either metabolized by the liver or released into the general circulation. TRANSPORT OF AMINO ACIDS INTO CELLS The concentration of free amino acids in the extracellular fluids is significantly lower than that within the cells of the body. This concentration gradient is maintained because active transport systems, driven by the hydrolysis of ATP, are required for movement of amino acids from the extracellular space into cells. At least seven different transport systems are known that have overlapping specificities for different amino acids. The small intestine and the proximal tubule of the kidney have common transport systems for amino acid uptake; therefore, a defect in any one of these systems results in an inability to absorb particular amino acids into the gut and into the kidney tubules. For example, one system is responsible for the uptake of cystine and the dibasic amino acids, ornithine, arginine, and lysine (represented as ―COAL‖). In the inherited disorder cystinuria, this carrier system is defective, and all four amino acids appear in the urine Amino acid deamination The first reaction in the breakdown of an amino acid is almost always removal of its alpha- amino group with the object of excreting excess nitrogen and degrading the remaining carbon skeleton or converting it to glucose. Urea, the predominant nitrogen excretion product in terrestrial mammals, is synthesized from ammonia and aspartate. Both of these latter substances are derived mainly from glutamate, a product of most deamination reactions. Most amino acids are deaminated by transamination, the transfer of their amino group to an alpha-keto acid to yield the alpha-keto acid of the original amino acid and a new amino acid,in reactions catalyzed by aminotransferases. REMOVAL OF NITROGEN FROM AMINO ACIDS A. Transamination: The funneling of amino groups to glutamate The first step in the catabolism of most amino acids is the transfer of their α-amino group to α-ketoglutarate(Figure 19.7). 4 | P a g e The products are an α-keto acid (derived from the original amino acid) and glutamate. α- Ketoglutarate plays a pivotal role in amino acid metabolism by accepting the amino groups from most amino acids, thus becoming glutamate. Glutamate produced by transamination can be oxidatively deaminated or used as an amino group donor in the synthesis of nonessential amino acids. This transfer of amino groups from one carbon skeleton to another is catalyzed by a family of enzymes called aminotransferases (formerly called transaminases). These enzymes are found in the cytosol and mitochondria of cells throughout the body—especially those of the liver, kidney, intestine, and muscle. All amino acids, with the exception of lysine and threonine, participate in transamination at some point in their catabolism. 1. Substrate specificity of aminotransferases: Each aminotransferaseis specific for one or, at most, a few amino group donors. Aminotransferases are named after the specific amino group donor, because the acceptor of the amino group is almost always α-ketoglutarate. The two most important aminotransferase reactions are catalyzed by alanine aminotransferase (ALT ) and aspartate aminotransferase ( AST ), a. Alanine aminotransferase (ALT): ALT is present in many tissues. The enzyme catalyzes the transfer of the amino group of alanine to α-ketoglutarate, resulting in the formation of pyruvate and glutamate. The reaction is readily reversible. However, during amino acid catabolism, this enzyme (like most aminotransferases ) functions in the direction of glutamate synthesis. Thus, glutamate, in effect, acts as a ―collector‖ of nitrogen from alanine. 5 | P a g e b. Aspartate aminotransferase (AST): AST is an exception to the rule that aminotransferases funnel amino groups to form glutamate. During amino acid catabolism, AST transfers amino groups from glutamate to oxaloacetate, forming aspartate, which is used as a source of nitrogen in the urea cycle 2. Mechanism of action of aminotransferases: All aminotransferasesrequire the coenzyme pyridoxal phosphate (a derivative of vitamin B6), which is covalently linked to the ε-amino group of a specific lysine residue at the active site of the enzyme. Aminotransferases act by transferring the amino group of an amino acid to the pyridoxal part of the coenzyme to generate pyridoxamine phosphate.
Details
-
File Typepdf
-
Upload Time-
-
Content LanguagesEnglish
-
Upload UserAnonymous/Not logged-in
-
File Pages14 Page
-
File Size-