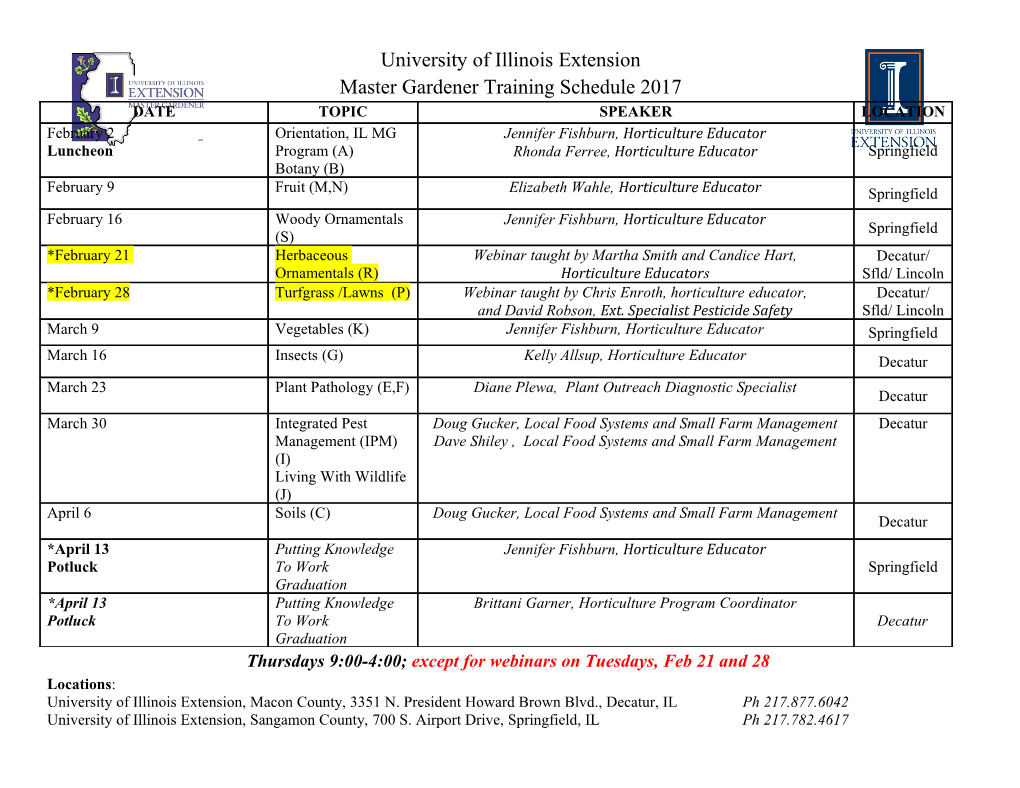
Vol. 134 (2018) ACTA PHYSICA POLONICA A No. 2 Quasi Fermi Level Scan of Band Gap Energy in Photojunction B.A. Orłowskia;∗, K. Gwóźdźb, M. Galickaa, S. Chusnutdinowa, E. Placzek-Popkob, M.A. Pietrzyka, E. Guziewicza and B.J. Kowalskia aInstitute of Physics, Polish Academy of Sciences, Aleja Lotnikow 32/46, PL-02668 Warsaw, Poland bDepartment of Quantum Technology, Faculty of Fundamental Problems of Technology, Wroclaw University of Science and Technology, Wybrzeze Wyspianskiego 27, 50-370 Wroclaw, Poland (Received April 9, 2018; in final form June 28, 2018) Photovoltage generation model results are compared with the correlated illumination intensity spectra of semiconductors photojunction. The moderate continuous increase of illumination intensity of semiconductor pho- tojunction leads to remarkable increase of relative concentration of minority carriers and related to it quasi Fermi level scan along the energy band gap. The scanning energy region runs up from thermal equilibrium Fermi level for electrons and down for holes. For moderate illumination related changes of quasi Fermi levels energy of mi- nority carriers dominate over the changes of majority carriers and they decide on measured open circuit voltage. Expected spectrum of quasi Fermi level scan on illumination intensity will strongly depend on interaction with electronic “defects” located in photojunction region (e.g. impurities, clusters, barriers, etc.) leading to the major- ity quasi Fermi level pinning. Measured region of quasi Fermi level energy pinning allows to estimate the defect states parameters (binding energy and concentration) in situ during the work of photojunction. The theoretical model of described effect will be presented and supported by experimental data measured for Si p=n junction and CdTe/ZnTe heterojunction. DOI: 10.12693/APhysPolA.134.590 PACS/topics: 73.40.Lq 1. Introduction structures, different electronic band structures, and dif- ferent minority and majority carriers at either side. Let Continuously ongoing advanced studies aimed at in- us assume that under illumination the same density of depth understanding of the physics of photovoltaic het- n and p carriers is generated at each illuminated point erojunctions [1–9] and improvement of construction of of heterojunction. Even so, this leads to a remark- solar cells [10–15] and ultraviolet sensitive photodetec- ably higher relative increase of concentration for minority tors [16–18] are in line with the search of renewable en- than for majority carriers and consequently to a different ergy sources supporting the sustainable civilization devel- changes of the corresponding quasi Fermi levels energies. opment. The comprehensive theory of solar cells exists Under illumination, the differences of the change of quasi and can be found in textbooks [3–6] convenient models Fermi energies for the same type of carriers (electrons or describing particular aspects of electricity generation in holes) contributes to create total photovoltage. a solar cell, facilitating experimental data interpretation The first part of the paper discusses photovoltage gen- or useful as an enlightening example are still welcome. eration in terms of basic correlations between the main fundamental parameters, such as: the changes of minor- In this paper we propose a model describing effect ity and majority carriers concentration, and the related of photovoltage generation by the analysis of the quasi changes of quasi Fermi energies of minority and major- Fermi level shifts induced by properly selected parame- ity carriers. The second part illustrates the correspon- ters of illumination (lasers beam with properly selected dence between the predicted open circuit photovoltage hν and intensity). It is also demonstrated that the model spectra and the results of corresponding experiments, can be used for interpretation of the data derived from with deviations caused by defects in the semiconductors the open circuit voltage measurements. Limitations re- junction region. lated to the presence of electrically active defects in the cell can easily be revealed by comparison of the data with the results of model calculations. 2. The model presentation Photovoltaic heterojunctions are built of two kinds of semiconductor materials with two kinds of electrons and The heterojunction of two semiconductors with differ- holes electronic structure in the conductions and valence ent band gaps, band offsets and type of carriers were an- bands on each side of the junction. In general, we have a alyzed in many works, like those of Fan [1], Anderson [3] junction of two semiconductors with different crystalline or Sze [4]. Figure 1 presents a simplified picture of the changes of quasi Fermi levels of minority and majority carriers relatively to the thermal equilibrium Fermi level energy, just to concentrate attention on changes of these ∗corresponding author; e-mail: [email protected] parameters under illumination. The region of the junc- tion is depicted by a broken line, and can be deformed (590) Quasi Fermi Level Scan of Band Gap Energy in Photojunction 591 by particular material parameters and defects occurring By analogy, the shifts of quasi Fermi levels of electrons during the growth processes. It will allow to study the and holes in side 1 (under illumination with hν > Eg1) influence of particular e.g. nano defects introduced to the are F1n and F1p, respectively, for electrons and holes (see junction region and compare it with spectra predicted by Fig. 1, side 1). Each relative change of the minority or the model for the parameters of these particular defects. majority carriers concentration is described by the cor- In the n-type semiconductor (side 1) the thermal equi- responding shifts of their quasi Fermi levels. In conse- librium Fermi level is located far from the valence band quence, under proper illumination of both sides of the edge, whereas in the p-type semiconductor (side 2) the F heterojunction, there will be four values of steady state level is located far from the conduction band edge. Un- quasi Fermi energies: F1n, F1p, F2n, and F2p. der illumination, the scan of quasi Fermi level of minor- The difference of the electric potential on the two ity carriers can occur in the wide energy range of related sides of the heterojunction for the electrons in conduc- band gaps. tion bands can be expressed by the difference of electrons quasi Fermi level shifts Let us consider the case when the incident light of V = (F − F )=e; Eg2 < hν < Eg1 is absorbed only at the side 2 of the n 2n 1n junction. Illumination of part 2 destroys the thermal where e is the electron charge. equilibrium condition of carriers and changes it to the For holes in the valence bands the electric potential steady state condition. The equal numbers of electrons difference will amount to and holes generated in part 2 increase the minority elec- Vp = (F1p − F2p)=e: tron concentration in the conduction band from n20 to n21 and the majority holes concentration in the valence The sum of the voltage Vn and Vp contributes to the total band from p20 to p21. To describe the electron concen- potential difference Vnp with the same polarity and with tration in part 2 a quasi Fermi level energy for electrons the same direction of the electric field. The direction of has to be introduced. This quasi Fermi level energy is the electric field (from side 1 to side 2) is determined shifted upwards relative to F by a value of F2n (see Fig. 1, by chemical potential differences in accordance with the side 2), adequate to the change of electron concentration. Fermi–Dirac function: positive for side 1 and negative for Similarly, for the holes generated in the valence band of side 2. The free electrons and holes will be separated and side 2 a quasi Fermi level energy has to be introduced to the measured photo cell voltage will be minus on side 1 shift down F by F2p (see Fig. 1, side 2). and plus on side 2. Fig. 2. The changes of quasi Fermi level positions cor- responding to the sequential increase of generated carri- Fig. 1. Illustration of quasi Fermi levels changes in a ers. For side 1 sequential increase of holes concentration schematic structure of a photovoltaic heterojunction. F from p10 to p11, p12 and p13 and electrons concentration is the thermal equilibrium Fermi energy, F1p and F1n are from n10 to n11, n12 and n13 leads to the change of quasi relative changes of quasi Fermi levels of minority holes Fermi levels energies of holes (minority carriers) to F1p1, p and majority electrons n after generation of n = p F1p2 and F1p3, and of electrons (majority) to F1n1;2;3. carriers on side 1 of the junction (n-type, wide band Analogical changes will appear for side 2 with minority gap Eg1), whereas F2n, F2p are the changes of quasi electrons and majority holes. The Voc in Fig. 2 neglects Fermi levels of minority electrons n and majority holes majority carriers contribution. p on side 2 (p-type, medium band gap Eg2) [8]. 592 B.A. Orłowski et al. 3. Change of carriers concentration The dependence of the quasi Fermi level energy on mi- and of quasi Fermi levels nority carriers concentration is presented in Fig. 3. The As already mentioned, in the photovoltaic effect the quasi Fermi level scan along forbidden gap leads to the change of carrier concentration leads to a corresponding change of open circuit voltage generation (Figs. 2 and 3). change of the quasi Fermi level and, consequently, to a The defect centers N1d and N2d located at the band gap change of the created open circuit photovoltage. The scan region can act as recombination centers and reduce changes are described by the Fermi–Dirac function or by the concentration of generated minority carriers p1 and the Maxwell–Boltzmann function — as an approximation n2 on side 1 and side 2, respectively.
Details
-
File Typepdf
-
Upload Time-
-
Content LanguagesEnglish
-
Upload UserAnonymous/Not logged-in
-
File Pages6 Page
-
File Size-