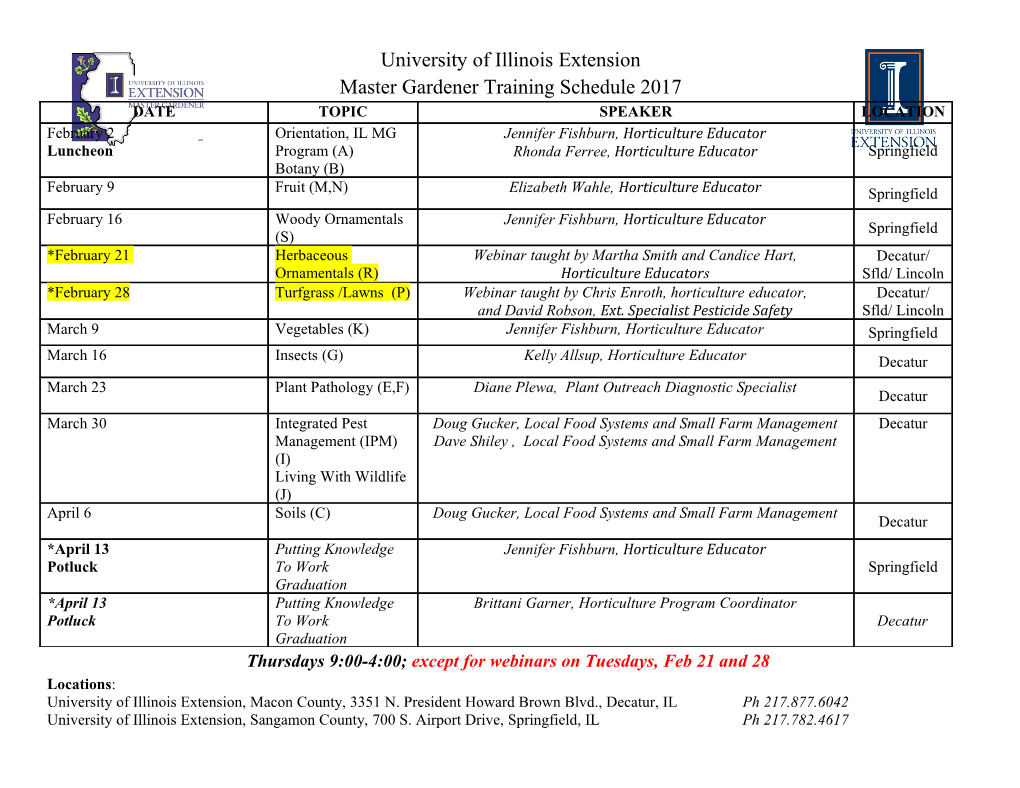
Advanced Space Propulsion Systems Content • Propulsion Fundamentals Advanced Space Propulsion Systems • Chemical Propulsion Systems • Launch Assist Technologies Lecture 317.014 • Nuclear Propulsion Systems • Electric Propulsion Systems • Micropropulsion Dr. Martin Tajmar • Propellentless Propulsion Institute for Lightweight Structures and Aerospace Engineering Space Propulsion, ARC Seibersdorf research • Breakthrough Propulsion 2 History & Propulsion Fundamentals Propulsion Fundamentals – 1.1 History Isaac Newton’s • Feng Jishen invested Fire Arrow in 970 AD Principia Mathematia (1687) • Used against Japanese Invasion in 1275 • Mongolian and arab troups brought it to Europe • 1865 Jules Verne published Voyage from Earth Reaction Principle to the Moon Constantin Tsiolkovski (1857-1935) Robert Goddard (1882-1945) Hermann Oberth (1894-1989) • Self-educated mathematics teacher • Launched first liquid-fueled • Die Rakete zu den m rocket 1926 Planetenräumen (1923) ∆v = v ⋅ln 0 • The Investigation of Space by rocket 1926 Planetenräumen (1923) p m Means of Reactive Drives (1903) • Gyroscope guidance • Most influencial on Wernher • Liquid-Fuel Rockets, multi-staging, patens, etc. von Braun 3 4 artificial satellites 1.1 History 1.1 History Fritz von Opel – RAK 2 (1925) Saturn V Treaty of Versailles from World War I Wernher von Braun prohibits Germany from Long-Range Space Shuttle Fritz Lang – Die Frau im Mond (1912-1977) Artillery • Hermann Oberth contracted to built rocket for premiere showing Walter Dornberger recruits Wernher von • Rocket was not finished, but key advancements A-4 / V-2 Braun from the Verein für Raumschifffahrt accomplished and movie was big success to develop missile in Penemünde (1932) • A4 (V2) first ballistic missile Sergei Korolev Valentin Glushko • A9/A10 on the drawing (1907-1966) (1908-1989) board (intercontinential Energia / Buran missile) 5 6 N-1 1 1.2 Propulsion Fundamentals 1.2.2 Delta-V Budget Most important to select propulsion system ! Conservation of Momentum ∆v = ∆vg + ∆vdrag + ∆vorbit − ∆vinitial v Gravitational Potential Drag Orbit Initial v dI d v p F = = m ⋅v = m ⋅v I = Force p p & p p Specific Impulse sp LEO 1.4 0.1 7.8 - 0.4 = 8.9 km/s dt dt g0 GEO 10.3 0.1 3 - 0.4 = 13 km/s dmp=dm0 dv dm p dm0 m0 ⋅ = ⋅v p dv = v p ⋅ dt dt m0 Mission Description Typical ∆v [km/s] LEO, GEO, Planetary Targets Satellites, Robotic missions 10-15 m dm0 Human Planetary Exploration Fast, direct trajectory 30 – 200 dv = v p ⋅ ∫∫m0 m0 100 – 1,000 AU (Distance Sun-Earth) Interstellar precursor mission 100 10,000 AU Mission to Oorth cloud 1,000 ∆v m0 Slow Interstellar 4.5 light-years in 40 years 30,000 Tsiolkovski ∆v = v ⋅ln m p = m0 ⋅ 1− exp − p v Equation m p Fast Interstellar 4.5 light-years in 10 years 120,000 7 8 1.2.2.1 Propulsion Requirements 1.2.2.1 Propulsion Comparison Max. Thrust [N] Saturn-V 7 10 Chemical Propulsion System Specific Maximum Maximum 6 Impulse [s] ∆v [km/s] * Thrust [N] 10 Nuclear Chemical Solid 250 – 310 5.7 – 7.1 107 5 10 MHD NERVA Liquid 300 – 500 6.9 – 11.5 107 4 MHD < 200 4.6 105 10 6 Nuclear Fission 500 – 800 11.5 – 20.7 10 103 Fusion 10,000 – 100,000 230 – 2,300 105 Antimatter 60,000 1,381 102 102 JIMO (Planned) Electric Electrothermal 150 – 1,200 3.5 – 27.6 101 1 Max. ∆v -1 10 Electric Electrostatic 1,200 – 10,000 27.6 - 230 3x10 [km/s] * 2 Photon Electromagnetic 700 – 5,000 16.1 – 115 10 * Assuming (m/m 7 -4 0 Propellantless Photon Rocket 3x10 unlimited 10 0 101 102 103 104 105 Exploratio Satellites Human Cloud Oorth Interstellar Slow Interstellar Fast Satellites Exploratio Human Breakthrough ? ? ? Cloud Oorth Interstellar Slow Interstellar Fast 0 )=0.1 * Assuming (m/m0)=0.1 ⇒ Spacecraft consists of 90% Propellant 9 10 n n 1.2.2 Single Staging – Multi Staging 1.2.2 Single Staging – Multi Staging Multi-Staging with separate structures, engines and tanks • Payload mass is directly linked to propellant velocity (e.g. Chemical 3,000-4,000, Electric up to 100,000) m0 mstructure + m propellant + m payload • Mass is directly linked to costs (e.g. 20 k$ / kg on Space Shuttle, 5 k$ on cheap = m m + 1−α ⋅ m + m m0 m0 Russian launcher) 1 structure ()propellant payload ln + ln ∆v1+2 m 1 m 2 = v p ⋅ 1−α ⋅ m + m + m ∆v m m0 ( ) [ structure propellant ] payload ln 0 = m m 2 ()1−α ⋅ mstructure + mpayload • Example for 100 kg rocket of mpropellant : mstructure : mpayload = 90 : 9 : 1 kg • Two stage rocket has maximum velocity gain of 1.43 at stage separator percentage of 91% • More stages increase velocity gain Structural Factor: Ratio of Empty Rocket (Structure + Payload) to Full Rocket (Structure + but also increases complexity Payload + Propellant) • Multi-Staging discovered by Calculated for Orbital Speed (∆v = 8,000 m/s, Atmospheric Drag + Gravity 1,500 – 2,000 m/s) Tsiolkovski in 1924 article Cosmic Single Stage Chemical Propulsion System (3,500 m/s) needs 90% propellant ! 11 Rocket Trains 12 2 1.2.2 Single Staging – Multi Staging (Saturn V) 1.3 Trajectory and Orbits Stage 1 Stage 2 Stage 3 Kepler laws are first correct description of the planet’s motion around the Sun Launch mass 2,286,217 kg 490,778 kg 119,900 kg Dry mass 135,218 kg 39,048 kg 13,300 kg G ⋅ M d m⋅ r − rΘ& 2 = − mr 2Θ& = 0 (&& ) 2 ( ) Propellant LO2/Kerosene LO2/LH2 LO2/LH2 r dt Balance of gravitational Conservation of angular Propellant velocity v 2,650 m/s 4,210 m/s 4,210 m/s p Tycho Brahe Johannes Kepler and centrifugal forces momentum Mass ratio 3.49 2.63 1.81 (1546-1601) (1571-1630) Velocity increment ∆v 3,312 m/s 4,071 m/s 2,498 m/s 1 GM rv2 • Parabola trajectory = ⋅()1+ ε ⋅cosΘ ε = −1 2 2 leaves Earth r r v GM 2GM v = r • Just √2 greater than circle (minimum orbit) – Mach 24 • Hyperbola trajectory used for interplanetary 13 flights 14 1.3.1 Keplerian Orbital Elements 1.3.2 Orbit Types Altitude Orbital Parameters Application Low Earth Orbit < 1000 km - Space Shuttle, Space (LEO) Station, Small Sats Geostationary Orbit 42,120 km i = 0° Communications (GEO) Molniya Orbit 26,600 km e = 0.75 Communication, i = 28.5 ° / 57 ° Intelligence Sun Synchronous 6,500 – 7,300 km i = 95 ° Remote Sensing Orbit • Semi-Major Axis a : size of elliptical orbit • Eccentricity ε : shape of orbit • Inclination I : angle of orbit with the equatorial plane • Longitude of ascending node Ω : inclination around semi-major axis a • Argument of perigee ω : Angle between ascending node and perigee Molniya Orbit • True anomaly ν : Angle between perigee and the spacecraft’s location 15 16 1.3.3 Orbit Transfers 1.4 Classification of Propulsion Systems • Hohmann Transfer Orbit: Most common and fuel efficient Internal Energy External Energy External / Internal Energy (example GTO-GEO) • Low Thrust Transfer Orbit: Internal Chemical Electric Nuclear (Induction Heating) Propellant E.g. Electric propulsion or solar sails External Air Breathing Propellantless Propellant MHD (Laser, Solar Sail) Ext. / Int. Air Breathing Propellant No Propellant Propellantless Propellantless (Solar Sail) Breakthrough Propulsion (Photon, Nuclear) Catapults • Gravity Assist Trajectory • Aerobrake Trajectory 17 18 3 Chemical Propulsion Systems 2. Chemical Propulsion Systems Combustion Exit Chamber Plane Thermodynamic Characterization Egas = c p ⋅ mp ⋅()Tc −Te Specific Heat at Constant Pressure Equal Kinetic Energy v p = 2⋅c p ⋅()Tc −Te Specific Heat: 1.2 – 1.3 γ /(γ −1) γ R Classical Gas Theory T ⋅ p = constant c p = ⋅ ()γ −1 mgas • pe/pc influenced by nozzle and Propellant Velocity atmosphere • T is function of chemical ()γ −1 /γ c 2γ R ⋅T p energy release c e vp = ⋅ ⋅ 1− • m small ⇒ high specific ()γ −1 mgas pc gas 19 impulse but low thrust 20 2.2 Liquid Propulsion Systems 2.2 Liquid Propulsion Systems • Liquid propellant stored in tanks – also mixture of liquid/solid called slush Fuel Oxydizer Average Density Specific Impulse • Fed into combustion chamber by pressurized gas or pump [g/cm3] [s] Kerosine (RP-1) Oxygen (O ) 1.02 300 – 360 Monopropellant Engines Used for Propellant 2 Hydrogen (H2) Oxygen (O2) 0.35 415 – 470 Unsymmetrical Nitrogen 1.20 300 – 340 Dimethyl Tetroxide (N O ) • Widely used for • Hydrazin (N2H4) 2 4 spacecraft attitude • Hydrogen Hydrazin (UDMH) and orbit control Hydrogen (H ) Fluorine (F ) 0.42 450 - 480 Peroxide (H2O2) 2 2 Due to catalyzer ⇒ low pressure required Propellant Combination Examples ⇒ low Isp of 150 – 250 s Bipropellant Engines • Used for launchers • Large variety and spacecraft available (LO - 2 Largest ever produced engines are the F-1 primary propulsion LH , …) Largest ever produced engines are the F-1 2 (Saturn V) and the RD-170 (Energia) systems Either separate plug is needed or propellants ignite at contact (hypergolic – like in Space Shuttle) 21 Saturn V – F1 22 General Dynamics / R-6 Rocket Engine General Dynamics / R-6 Rocket Engine • Thrust: 22 N (6.2 – 32.9 N) 31 N • Isp=290 s at 22 N • N2O4 (Nitrogen Tetraoxyde)– MMH (Monomethyl Hydrazine) • O/F Ratio = 1.65 35.6 N 35.6 N 24 bar ()γ −1 /γ 2γ R ⋅Tc pe 1370 °C v = ⋅ ⋅ 1− p γ −1 m p ()gas c 650 °C 23 49 °C 24 4 2.2.2/3 Solid / Hybrid Propulsion Systems 2.3 Nozzle Design Solid Propulsion Systems • Fuel and oxidizer are stored as grains Most common nozzles: Cone Nozzle Bell Nozzle glued together forming a kind of rubber • Typically hydrocarbon (fuel) and Most simple Reduces Beam Divergence ammonium perchlorate (oxidizer) • 16-18% of aluminium powder added to In atmosphere, the outside increase temperature and specific impulse pressure is balanced everywhere but on the nozzle exit Cons Pros • Can not be stopped • Very simple,
Details
-
File Typepdf
-
Upload Time-
-
Content LanguagesEnglish
-
Upload UserAnonymous/Not logged-in
-
File Pages26 Page
-
File Size-