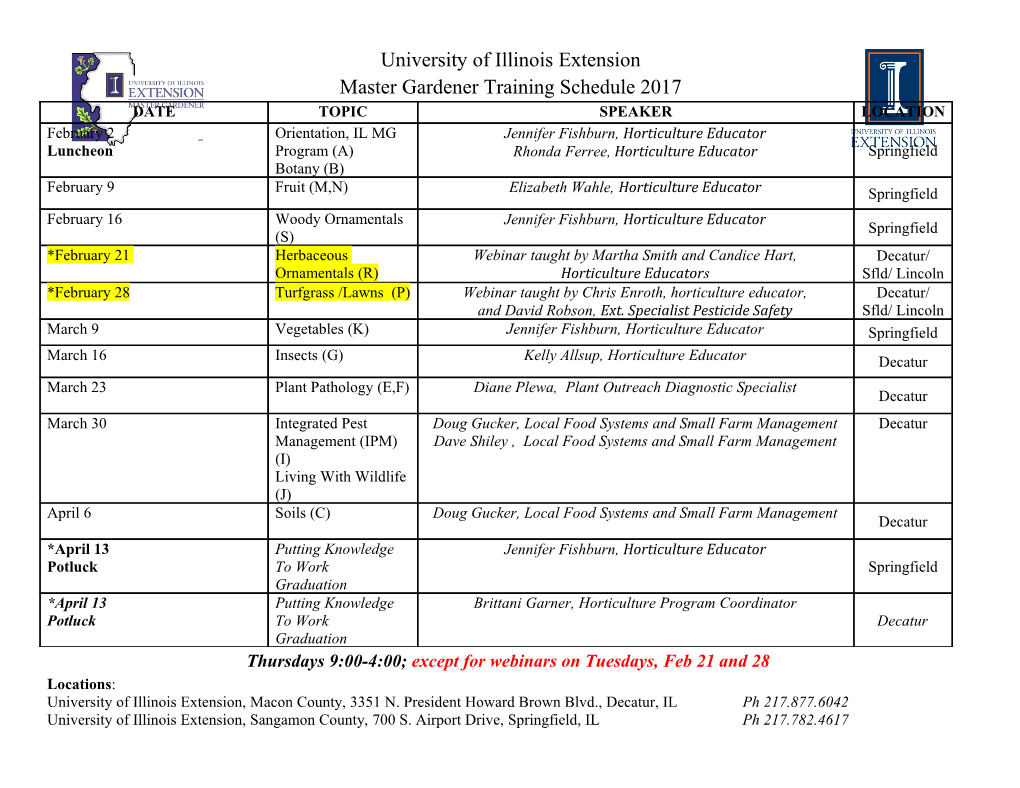
“Development of 3-D divertor solutions for stellarators through coordinated domestic and international research” Oliver Schmitz1 on behalf of U.S. stellarator collaborators* U.S. stellarator collaborators: * J.P. Allain3, D.T. Anderson1, A.H. Boozer2, D. Curreli3, J.P. Freidberg4, D.A. Gates5, J.H. Harris6, S.R. Hudson5, M. Landreman7, J.D. Lore6, G.H. Neilson5, D.A. Maurer8, A. Reiman5, D. Ruzic3, D.A. Spong6,J.N. Talmadge1, F.A. Volpe2, H. Weitzner9, G.A. Wurden10, M.C. Zarnstorff5 1 – University of Wisconsin 6 – Oak Ridge National Laboratory 2 – Columbia University 7 – University of Maryland 3 – University of Illinois 8 – Auburn University 4 – Massachusetts Institute of Technology 9 – New York University 5 – Princeton Plasma Physics Laboratory 10 – Los Alamos National Laboratory I.) Introduction: This paper, a supplement to the stellarator community white paper and presentations, describes a set of three initiatives aiming on development of a 3-D divertor solution for stellarators within the next decade. Development of a 3-D divertor solution for Long Pulse Burning Plasma science is a key challenge on the way to qualify the stellarator as a potential candidate for a demonstration fusion reactor.. Optimization of 3-D confinement and 3- D equilibrium properties in stellarators go hand-in-hand with the development of a viable 3-D divertor solution in an integrated approach for confinement of stationary, disruption free, high performance plasmas. A coherent initiative is proposed to enhance international stellarator collaboration towards this mission goal and link it to a thriving, well-targeted domestic research program. Our aim is to expand on our strong standing in the field and take up leadership on an international scale. Key issues which have to be addressed for the development of a credible 3-D divertor solution are (a) a divertor structure that is robust to finite beta and bootstrap current effects on the equilibrium (b) the ability to handle the heat exhaust through a combination of radiation with detachment (partial or full) and expanded target surface area (c) capability to reduce neutral recycling and impurity influx from the wall to the plasma edge and (d) compatibility to core transport performance which is intrinsically bound in stellarators to the 3-D equilibrium choice (optimization route). The issue of a robust divertor with respect to stability of the magnetic configuration concerns the residual plasma currents existing even in optimized stellarators. These currents change the rotational transform and hence the edge magnetic field structure employed as divertor. This has consequences for the heat and particle loads of divertor and first wall elements as well as on the particle exhaust properties. Today two concepts are used - the helical divertor at the Large Helical Device in Japan and the island divertor at Wendelstein 7-X in Germany. Both are quite different concepts, but both require a stable edge magnetic topology to work as feasible 3D divertor concepts. There are significant concerns as far as the internal plasma response (in particular, the residual bootstrap and Pfirsch-Schlüter currents) interacts with the divertor structures. The edge magnetic field defines the neutral and impurity transport as well as the particle and heat exhaust characteristics. Hence, there is a direct link established between the internal plasma currents and the plasma edge transport and plasma wall interaction. Reducing and controlling the level of internal currents is a means to control the plasma edge features. At the same time, the transport across good flux surfaces in the plasma defines the particle and heat inflow into the plasma boundary and hence the resulting exhaust requirement. This points out that development of a 3-D divertor solution in stellarators requires taking plasma transport inside of the last closed flux surface into account. The edge structure will determine the inward transport of neutrals and impurities and hence – in an ion root transport regime (i.e. a negative radial electric field) – also the level of impurity accumulation and helium retention in the core. Consequently, core transport phenomena are directly linked to the optimization of the edge magnetic field structure. This sequence of key issues of our development strategy for a 3-D divertor solution shows that we seek to setup an integrated approach, which concentrates on the 1 3-D divertor structure and related edge transport and plasma wall interaction. However, with a suite of diagnostics and modeling tools we will assess at the same time how feasible a given 3-D divertor concept is as an integrated component of a long pulse plasma including plasma core transport and performance aspects. The goals and means of these initiatives are shown in figure 1. The goal of these initiatives is to develop a credible 3-D divertor solution for stellarators through a coordinated research effort internationally and on domestic experiments. The initiatives are described in the following section II with the three budget scenarios stated in the charge to FESAC discussed at the end of each initiative. Concluding remarks addressing prioritization are described in section III. Figure 1: Goals and means of initiative on 3D divertor physics in stellarators II.) Initiatives Initiative I: Enhance international stellarator collaboration enabling access and leadership participation on forefront, large-scale stellarator facilities Two large-scale superconducting devices constitute the experimental basis of the international stellarator collaboration program - the LHD stellarator in Japan and the Wendelstein 7-X (W7- X) stellarator in Germany. Both devices feature quasi-stationary plasmas with specific divertor concepts which have to be qualified as viable divertor solutions. At LHD, recently a closed helical divertor has been installed. The divertor structure consists of a stochastic magnetic field layer in the very edge and long divertor legs in which the magnetic field lines approach the divertor surfaces under very shallow angles. This first of its kind setup allows the testing of the quasi-stationary particle and heat exhaust properties. This setup is in strong contrast to the W7-X island divertor as shown in figure 2. Figure 2: Plasma edge structure of the helical divertor at LHD, NIFS, Japan on the left and the modular island divertor structure at Wendelstein 7-X, MPI-IPP, Germany on the right. 2 Here, magnetic islands in the plasma edge are used to form the divertor structure. Comparing both divertor geometries and qualifying them towards heat and particle exhaust properties as well as impurity retention and density control is a unique opportunity with W7-X coming online at the same time LHD is operating as a mature large scale stellarator device. The LHD directorate has endorsed participation of U.S. scientists and recently intensified the encouragement to participate in LHD research. We have already provided a crystal soft x-ray imaging spectrometer and a strong collaboration path exists. The U.S. XICS system provides an available opportunity for the U.S. to participate in their exciting plans for the next several years, including their new divertor configuration and long-awaited deuterium plasma operation. On W7-X, we are particularly interested in 3-D edge plasma/divertor physics as a key aspect of an integrated physics program including 3-D core transport and plasma control in long pulse operation. We have provided trim coils, which are an actuator for fine-tuning the edge magnetic field structure and hence are a key tool to systematically change divertor topology. The ongoing design of an active protection element, or so-called scraper element, for critical high heat flux divertor regions has been driven by U.S. involvement and in concert with the trim coils and current US diagnostic activities on W7-X provides for an excellent basis for a U.S. leadership role in establishing the island divertor physics basis for successful W7-X operations. Moreover, this particular activity aids generic diagnostic and hardware developments for other long pulse devices like surface heat load control by IR spectroscopy and pellet fueling at ITER. A recent workshop on possible university contributions to W7-X has highlighted, that a very strong alignment exists between W7-X programmatic needs and U.S. University and national laboratory activities and expertise. A strong national lab programmatic setup is already in place and the Wendelstein 7-X directorate has strongly endorsed the U.S. collaboration in particular in the field of 3-D divertor physics as key to the success of the device. The U.S. research agenda for W7-X will make use of its contributions to date and will focus strongly on edge and divertor physics in its first years. The TDU scraper element, with its embedded diagnostics combined with U.S. infrared imaging instruments and U.S. modeling efforts, will enable measurements of edge plasma transport coefficients. Critical to these studies is the ability to control the edge magnetic field structure, including the use of U.S. trim coils to correct for error fields. The U.S. has the edge physics and magnetics modeling tools needed for these studies, which will provide in addition opportunity for validation. Investigation of the edge cannot be decoupled from understanding of the core and the U.S. will use its XICS to contribute with temperature and velocity profile measurements of the core. Over time, U.S. core physics research on W7-X will grow; plans include density profile control with pellet fueling, energetic particle physics studies, and transport physics studies with fluctuation diagnostics. U.S. participation in both the LHD and W7X world-class stellarator programs will enable advances in understanding through comparison based on their similarities and differences, as well as model validation against data from multiple sources. The planned U.S. activities on both devices have been aligned with the programmatic interests of the local scientific team and other international collaborators.
Details
-
File Typepdf
-
Upload Time-
-
Content LanguagesEnglish
-
Upload UserAnonymous/Not logged-in
-
File Pages10 Page
-
File Size-