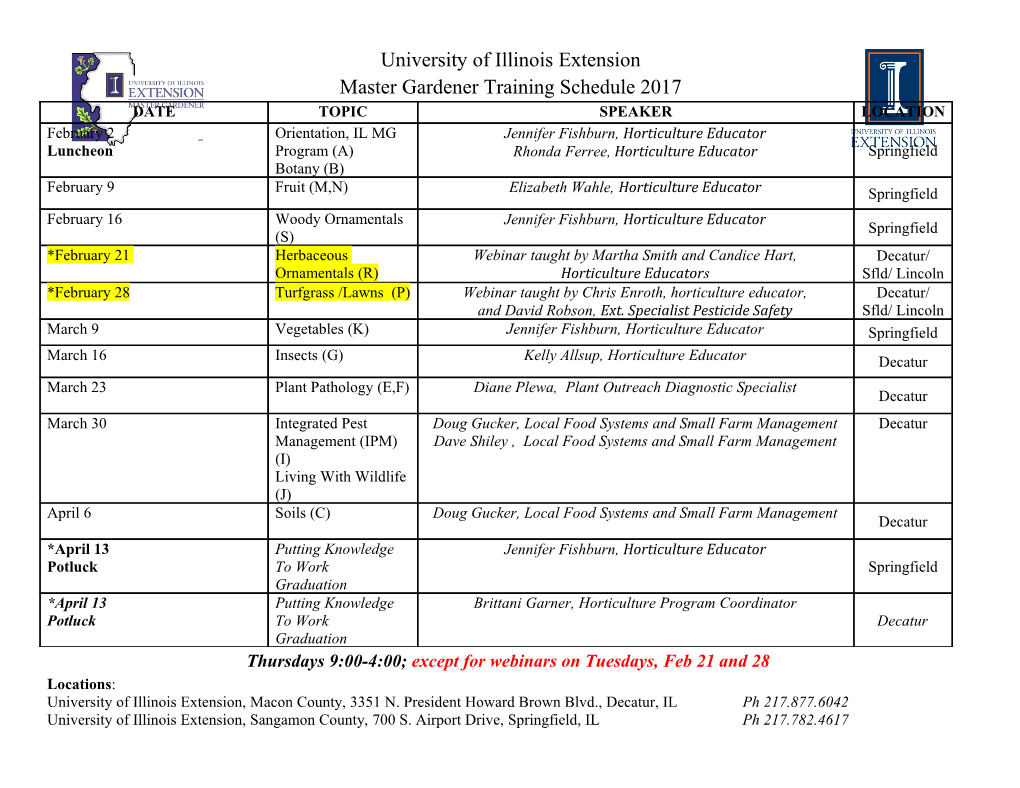
Development and Characterisation of Polyhydroxybutyrate from Selected Bacterial Species By Soroosh Bagheriasl, MRes. A thesis submitted to the University of Birmingham for the degree of DOCTOR OF PHILOSOPHY SCHOOL OF METALLURGY AND MATERIALS UNIVERSITY OF BIRMINGHAM October 2012 University of Birmingham Research Archive e-theses repository This unpublished thesis/dissertation is copyright of the author and/or third parties. The intellectual property rights of the author or third parties in respect of this work are as defined by The Copyright Designs and Patents Act 1988 or as modified by any successor legislation. Any use made of information contained in this thesis/dissertation must be in accordance with that legislation and must be properly acknowledged. Further distribution or reproduction in any format is prohibited without the permission of the copyright holder. Abstract Polyhydroxyalkanoates (PHAs) are biodegradable, linear polyesters produced primarily by bacterial fermentation. PHAs have been found to possess similar properties to many non- biodegradable petrochemical plastics. The current cost of PHA production is far greater in comparison to petrochemical plastics. Recent research has focused on developing cost effective production of PHAs from various strains of bacteria. In this study Serratia sp. NCIMB 40259 and R. eutropha H16 were utilised for the accumulation of Polyhydroxybutyrate (PHB). Serratia sp. with high phosphatase activity (HPA) or low phosphatase activity (LPA) were fermented for 48, 120, 216 and 320 h and dosed daily with stock solutions in order to accumulate intracellular PHB and biomineralise hydroxyapatite (HA) on the cell surface. The optimum conditions for the accumulation of PHB, in this study, were found to be 216 h fermentation, producing a PHB yield of 14.6 %w/w and 3.3 %w/w for LPA and HPA respectively. The accumulation of PHB by Serratia sp. was found to be directly dependant on the fermentation time. The overall average yields of PHB from LPA Serratia cells were consistently higher than that from HPA cells. Phosphatase activity was observed to have an indirect effect on the accumulation of PHB. The molecular and thermal properties of PHB were analysed by means of Fourier Transform Infrared Spectroscopy (FTIR), Differential Scanning Calorimetry (DSC) and Gel Permeation Chromatography (GPC) and were observed to be largely unaffected by the phosphatase activity of the cells and appeared to be dependent on the fermentation period. A correlation was observed between the fermentation periods, Molecular Weight (Mw) and Polydispersity (PD). GPC analysis of PHB from HPA and LPA Serratia sp. showed similar Mw and PD values over the range of fermentation periods. The breadth of the melting endothermic peaks for PHB from LPA and HPA showed the same pattern of peak broadening with increasing fermentation time. This was attributed to the increase in PD and the presence of smaller polymer chain lengths that reach complete melt at varying temperatures. The % crystallinity of PHB from HPA and LPA were highest after 216 h fermentation and correlated with the peak intensities of the endothermic melting peak of the DSC traces. The % crystallinity was in the expected region for PHB. The mineralisation of HA was observed to be dependent on the fermentation period and phosphatase activity of the cells. A longer fermentation period yielded greater mineralisation of HA. The fermentations of LPA cells showed an initial decrease in HA mineralisation, while fermentations of HPA cells yielded the highest overall average yields of HA. The production of HA alongside PHB by Serratia sp. makes the process more economically viable. In order to make the process a viable option for the production of PHB, optimisation of the fermentation conditions for the accumulation of PHB is necessary, whilst still maintaining high yields of HA mineralisation. Th R. eutropha cells were fermented with the substrates olive oil and rapeseed oil and compared with glucose. Small scale fermentations using 20 g/l of oils (rapeseed or olive) and glucose, as carbon sources, were conducted. The use of either olive oil or rapeseed oil as carbon sources resulted in the greatest accumulation of PHB at 36 %w/v compared to 12 %w/v from glucose. The thermal and molecular properties of PHB from the various carbon sources were analysed by means of FTIR, DSC and GPC, and were found to be almost identical. The diameter of the electrospun nanofibres for PHB obtained from glucose (G-PHB), Olive oil (O-PHB) and Rapeseed oil (R-PHB) were found to be similar. This study shows that the source of carbon used did not have a significant effect on the diameters of electrospun nanofibres, this was attributed to the similar Mw properties of each carbon source. The electrospun fibres of G-PHB, O-PHB and R-PHB were found to have diameters dependant on their solution concentrations. Lower solution concentrations resulted in smaller diameter fibres. The use of oils in the production of PHB would make a viable replacement for the use of sugars as substrates. It has been suggested that rapeseed oil is a low cost alternative to other conventional oils and in this study rapeseed oil resulted in an almost identical yield of PHB compared with the more costly olive oil. By adjusting the electrospinning parameters it may be possible to control the crystallinity and the diameters the PHB fibres. Controlling the crystallinity and fibre diameters of PHB electrospun PHB is essential if they are to be used in biomedical applications. Acknowledgments It has been a great experience and honour to have carried out this project at The University of Birmingham. I would like to express my sincere thanks and appreciation to my supervisor, Dr Artemis Stamboulis, for all her inspirational mentorship throughout, from my initial Masters through to the completion of this project. I would also like to extend my gratitude to Dr Mike Jenkins, for his willingness to offer guidance and support. I would also like to give special thanks to Dr Iza Radecka, from the University of Wolverhampton. She was always willing to provide me with much appreciated guidance, and of course PHB, throughout the project. Additionally, I would like to thank Victor Irorere who contributed much of his time in assisting with the fermentation experiments. I would like to thank my colleagues within the biomaterials group. The steady friendship and support that they offered, has helped make my time at the University of Birmingham rewarding and memorable. In particular, I would like to thank Mark Blevins, without him the art of electrospinning may well have remained a mystery to me. By the same token, my family deserve equal accolade. The unwavering encouragement and reassurance that they provided has been integral in my completion of this degree. My amazing girlfriend has always managed to lift my spirit when I was down and I don’t think I would have finished this work without her constant support. Contents List of Figures .................................................................................................................................... iv List of Tables .................................................................................................................................... viii Abbreviations ......................................................................................................................... x Chapter 1 : Introduction and Literature Review .................................................................. 1 1.1. Introduction .................................................................................................................................. 2 1.2. Waste problems associated with the use of synthetic plastics ...................................................... 3 1.3. Polyhydroxyalkanoates (PHAs) ................................................................................................... 4 1.4. Physical, mechanical and chemical properties of poly-hydroxy-alkanoates ................................ 7 1.4.1. Chemical Structure of PHAs ................................................................................................. 7 1.4.2. The physical and mechanical properties of PHAs ............................................................... 10 1.4.3. Thermal properties of poly-hydroxy-alkanoates ................................................................. 13 1.4.4. Biodegradability of poly-hydroxy-alkanoates ..................................................................... 14 1.4.5. Biocompatibility of poly-hydroxy-alkanoates ..................................................................... 16 1.5. Bacteria producing Poly-hydroxy-alkanoates ............................................................................ 16 1.5.1. Ralstonia eutropha ............................................................................................................... 18 1.5.2. Serratia sp. ........................................................................................................................... 19 1.6. Inclusion body formation ........................................................................................................... 21 1.7. Bacterial synthesis pathway ....................................................................................................... 22 1.8. Applications of Bioplastics ........................................................................................................ 25 1.8.1. Advancements
Details
-
File Typepdf
-
Upload Time-
-
Content LanguagesEnglish
-
Upload UserAnonymous/Not logged-in
-
File Pages209 Page
-
File Size-