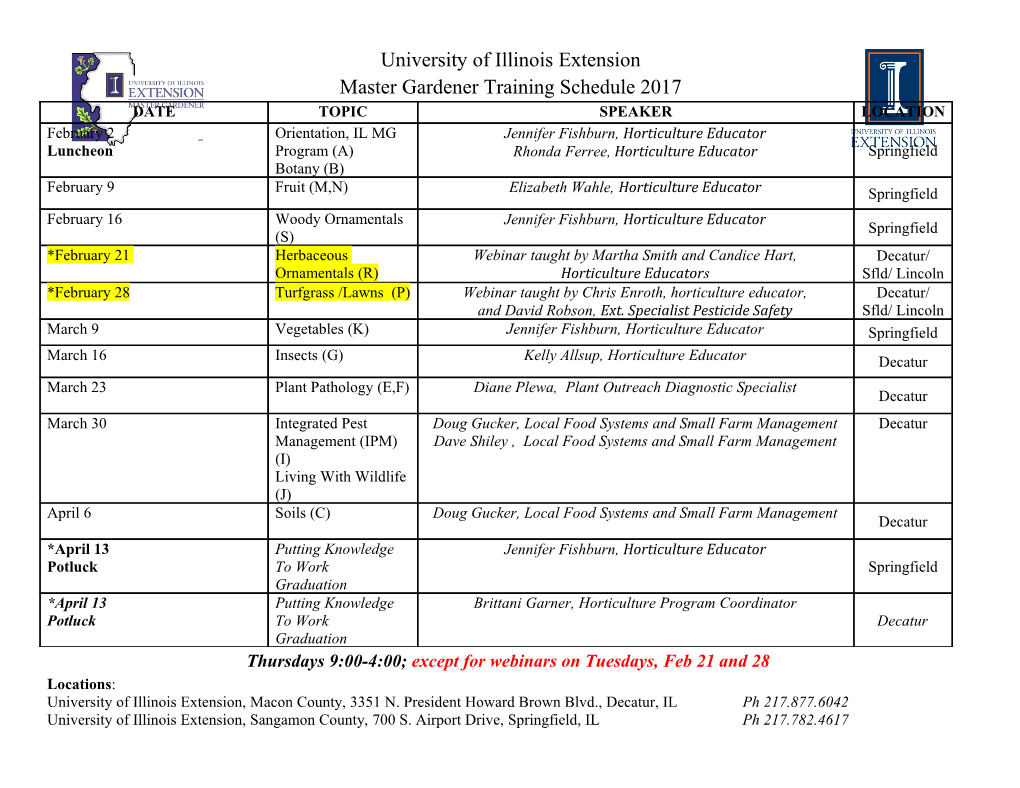
Cavity Optomechanics with Silica Toroidal Microresonators down to Low Phonon Occupancy R´emiRivi`ere M¨unchen2011 Cavity Optomechanics with Silica Toroidal Microresonators down to Low Phonon Occupancy R´emiRivi`ere Dissertation an der Fakult¨atf¨urPhysik der Ludwig{Maximilians{Universit¨at M¨unchen vorgelegt von R´emiRivi`ere aus Toulouse, Frankreich M¨unchen, den 29. August 2011 Erstgutachter: Prof. Dr. T. W. H¨ansch Zweitgutachter: Prof. Dr. J. P. Kotthaus Tag der m¨undlichen Pr¨ufung:30. September 2011 A mes parents, Christine et Jean et `ama soeur, Laura. Travaillez, prenez de la peine. C'est le fonds qui manque le moins. Le laboureur et ses enfants Jean de la Fontaine vi Contents List of Figures ix List of Publications xi List of Conference Talks xiii Zusammenfassung xv Abstract xvii 1 Theory of cavity optomechanics 1 1.1 Introduction ................................... 1 1.2 Theoretical framework of cooling ....................... 3 1.2.1 Hamiltonian of the optomechanical system .............. 4 1.2.2 Equations of motion .......................... 5 1.2.3 Static backaction ............................ 6 1.2.4 Dynamical backaction ......................... 6 1.2.5 Optical spring and damping effects .................. 9 1.2.6 Quantum backaction .......................... 10 1.2.7 Dynamical backaction cooling ..................... 10 1.2.8 Dynamical backaction heating and amplification . 13 1.2.9 The sideband picture - analogy with atomic cooling . 13 1.3 Detection sensitivity .............................. 16 1.3.1 Measurement imprecision and measurement backaction . 16 1.3.2 Total measurement uncertainty .................... 18 2 Silica toroidal microresonators as candidates for cavity optomechanics 21 2.1 Introduction ................................... 21 2.2 Optical modes in silica toroidal resonators . 23 2.2.1 Analytic derivation of optical modes in spheres . 23 2.2.2 Approximated derivation of optical modes in toroids . 24 2.2.3 Analysis of whispering-gallery mode losses in microtoroids . 25 2.2.4 Optical fiber taper coupling to optical resonator modes . 27 2.3 Mechanical modes in silica toroidal resonators . 30 viii CONTENTS 2.3.1 Supported mechanical modes in toroids . 30 2.3.2 Mechanical losses ............................ 32 2.4 Optomechanical coupling ............................ 34 2.4.1 Effective displacement ......................... 34 2.4.2 Effective mass .............................. 34 3 Optomechanical cooling to low phonon occupancy 37 3.1 Introduction ................................... 37 3.2 Reduction of the mechanical dissipation ................... 39 3.2.1 Gas damping .............................. 39 3.2.2 Clamping-induced damping ...................... 43 3.2.3 Material damping ............................ 49 3.3 The resolved-sideband regime ......................... 59 3.4 Reduction of the environmental temperature . 64 3.4.1 The prototype helium-4 cryostat .................... 64 3.4.2 Taper coupling at low temperature . 67 3.4.3 Thermalization ............................. 69 3.4.4 Optical cryogenic properties of silica microtoroids . 74 3.4.5 Thermal response ............................ 78 3.4.6 Dynamical backaction cooling in the helium-4 cryostat . 84 3.4.7 The experimental helium-3 cryostat . 87 3.5 Measurement of low phonon numbers ..................... 91 3.5.1 The balanced homodyne detection ................... 91 3.5.2 Dynamical backaction cooling ..................... 96 3.6 Optomechanically induced transparency . 102 3.6.1 Principle of the optomechanically induced transparency . 102 3.6.2 Optomechanically induced transparency measurements . 105 3.6.3 Analogy to atomic Electromagnetically Induced Transparency . 107 4 Summary and outlook 111 4.1 Summary of the doctoral work . 111 4.2 Outlook ..................................... 113 A Microfabrication of silica microtoroids 115 B Fabrication of tapered optical fibers 119 C Manipulation procedure of the prototype helium-4 cryostat 121 D Gallery of on-chip optical microstructures 125 Bibliography 127 Acknowledgements 143 List of Figures 1.1 Generic optomechanical system ........................ 3 1.2 Sideband picture of the optomechanical cooling . 15 1.3 Squared amplitude and phase of the output field relative to the input field. 17 1.4 Total uncertainty on the position measurement at zero detuning. 19 2.1 Scanning electron micrograph, scheme and optical micrograph of toroids . 24 2.2 Numerical simulation of the electric field in an intracavity whispering-gallery- mode ....................................... 26 2.3 Numerical simulation of mechanical modes in microtoroids . 32 3.1 Picture and 3D rendering of the vacuum chamber . 40 3.2 Experimental setup and principle of the side-of-the-fringe detection . 41 3.3 Mechanical quality factor versus pressure for a toroid and a disk . 42 3.4 Radial-breathing-mode mechanical Q and frequency versus relative undercut 44 3.5 Comparative mechanical Q and frequency versus relative undercut . 45 3.6 Scanning electron microscope picture of a needle-pillar supported structure 46 3.7 Scanning electron micrograph of a spoke toroid . 47 3.8 Microfabrication steps of a spoke toroid .................... 48 3.9 Measured mechanical Q versus parameter D and numerical simulation of the displacement for an optimized spoke toroid . 49 3.10 2D structure of crystalline and glassy SiO2 and two-level system configuration 51 3.11 Inverse mechanical Q and relative mechanical frequency shift down to 4He temperatures .................................. 53 3.12 Inverse mechanical Q and relative mechanical frequency shift down to 3He temperatures .................................. 56 3.13 Mechanical Q versus resonant driving ..................... 57 3.14 Normalized intracavity photon number versus resolved-sideband factor to obtain unity occupancy ............................. 60 3.15 Motional sidebands on the transmission spectrum of a toroidal cavity un- dergoing coherent mechanical oscillations ................... 62 3.16 Optical layout of the quantum-limited Ti:sapphire laser used in the experiment 63 3.17 Technical layout of the 4He cryostat ...................... 66 3.18 Picture and technical layout of the cryohead . 68 x LIST OF FIGURES 3.19 Picture and technical layout of the cryotaper . 69 3.20 Fabrication and installation steps of the cryotaper . 70 3.21 Pound-Drever-Hall detection scheme ..................... 72 3.22 Thermalization in the 4He cryostat ...................... 73 3.23 Optical resonance shift for varying temperatures down to 1:65 K . 75 3.24 Principle of bistable intracavity power .................... 77 3.25 Multistable intracavity power at low temperature . 78 3.26 Response measurement setup ......................... 81 3.27 Cryogenic response cutoff frequencies versus cryostat temperature . 82 3.28 Amplitude response in the presence of a film of superfluid 4He . 83 3.29 Cryogenic pre-cooling and laser cooling .................... 85 3.30 Quantification of intracavity absorption heating . 86 3.31 3He exchange gas cryostat and cryohead ................... 88 3.32 Mechanical radial breathing mode thermalization curve in the 3He cryostat 89 3.33 Simplified scheme of the balanced homodyne detection principle . 93 3.34 Optical and electronic layout of the Ti:sapphire laser and the balanced ho- modyne detection ................................ 94 3.35 Optical spring and damping effects. ...................... 97 3.36 Cooling factor and phonon occupancy versus laser detuning . 99 3.37 Optomechanically induced transparency principle. 103 3.38 The classical optomechanical system . 104 3.39 Optomechanically induced transparency measurements . 106 3.40 Power dependence of the transparency parameters . 107 3.41 The coherent population trapping example . 108 3.42 Atomic EIT - OMIT energy level analogy . 109 A.1 Microfabrication steps of the toroidal microcavities . 116 C.1 Photographs of the cryostat and the cryoprobe . 123 C.2 Photographs of the opened prototype cryostat . 123 D.1 On-chip silica optical delay elements . 125 D.2 Spoke silica toroidal and disk microresonators . 125 D.3 Spoke silica toroidal microresonator . 126 List of Publications [1] A. Schliesser, R. Rivi`ere,G. Anetsberger, O. Arcizet, and T. J. Kippenberg \Resolved-Sideband Cooling of a Micromechanical Oscillator" Nature Physics 4, 415 (2008). [2] G. Anetsberger, R. Rivi`ere,A. Schliesser, O. Arcizet, and T. J. Kippenberg \Ultralow-Dissipation Optomechanical Resonators on a Chip" Nature Photonics 2, 627 (2008). [3] A. Schliesser, G. Anetsberger, R. Rivi`ere,O. Arcizet, and T. J. Kippenberg \High-Sensitivity Monitoring of Micromechanical Vibration using Optical Whispering Gallery Mode Resonators" New Journal of Physics 10, 095015 (2008). [4] O. Arcizet∗, R. Rivi`ere∗, A. Schliesser, G. Anetsberger, and T. J. Kippenberg \Cryogenic Properties of Optomechanical Silica Microcavities" Physical Review A 80, 021803 (2009). [5] A. Schliesser∗, O. Arcizet∗, R. Rivi`ere∗, G. Anetsberger, and T. J. Kippenberg \Resolved-Sideband Cooling and Position Measurement of a Micromechanical Oscillator Close to the Heisenberg Uncertainty Limit" Nature Physics 5, 509 (2009). [6] G. Anetsberger, O. Arcizet, Q. P. Unterreithmeier, R. Rivi`ere,A. Schliesser, E. M. Weig, J. P. Kotthaus, and T. J. Kippenberg \Near-Field Cavity Optomechanics with Nanomechanical Oscillators" Nature Physics 5, 909 (2009). [7] S. Weis∗, R. Rivi`ere∗, S. Del´eglise∗, E. Gavartin, O. Arcizet, A. Schliesser, and T. J. Kip- penberg \Optomechanically Induced Transparency" Science 330, 1520 (2010).
Details
-
File Typepdf
-
Upload Time-
-
Content LanguagesEnglish
-
Upload UserAnonymous/Not logged-in
-
File Pages162 Page
-
File Size-