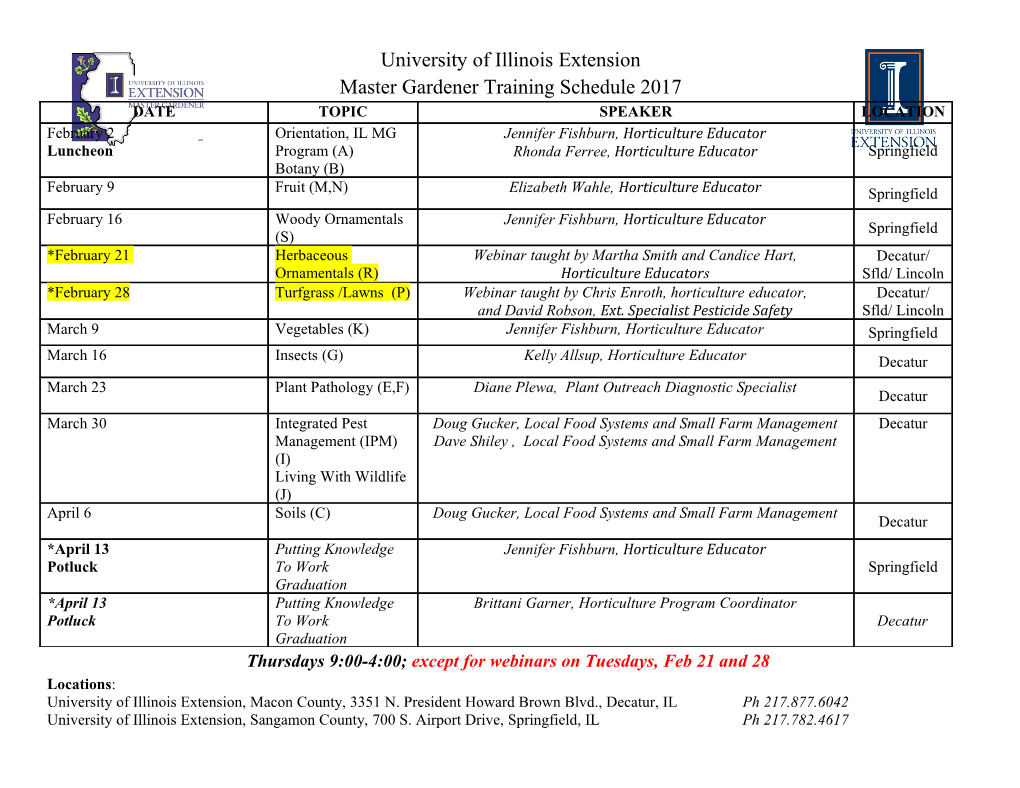
ANRV342-PP59-05 ARI 27 March 2008 1:28 Chlorophyll Fluorescence: A Probe of Photosynthesis In Vivo Neil R. Baker Department of Biological Sciences, University of Essex, Colchester, CO4 3SQ, United Kingdom; email: [email protected] Annu. Rev. Plant Biol. 2008. 59:89–113 Key Words The Annual Review of Plant Biology is online at carbon dioxide assimilation, electron transport, imaging, plant.annualreviews.org metabolism, photosystem II photochemistry, stomata This article’s doi: 10.1146/annurev.arplant.59.032607.092759 Abstract Copyright c 2008 by Annual Reviews. The use of chlorophyll fluorescence to monitor photosynthetic per- All rights reserved formance in algae and plants is now widespread. This review exam- 1543-5008/08/0602-0089$20.00 ines how fluorescence parameters can be used to evaluate changes by Miami University of Ohio on 09/23/10. For personal use only. in photosystem II (PSII) photochemistry, linear electron flux, and CO2 assimilation in vivo, and outlines the theoretical bases for the Annu. Rev. Plant Biol. 2008.59:89-113. Downloaded from www.annualreviews.org use of specific fluorescence parameters. Although fluorescence pa- rameters can be measured easily, many potential problems may arise when they are applied to predict changes in photosynthetic perfor- mance. In particular, consideration is given to problems associated with accurate estimation of the PSII operating efficiency measured by fluorescence and its relationship with the rates of linear electron flux and CO2 assimilation. The roles of photochemical and non- photochemical quenching in the determination of changes in PSII operating efficiency are examined. Finally, applications of fluores- cence imaging to studies of photosynthetic heterogeneity and the rapid screening of large numbers of plants for perturbations in pho- tosynthesis and associated metabolism are considered. 89 ANRV342-PP59-05 ARI 27 March 2008 1:28 cence changes in vivo are complex and cor- Contents rect interpretation of changes in fluorescence parameters can often be difficult. Considera- INTRODUCTION................. 90 tion is given to some problems associated with BACKGROUND ................... 90 the measurement of these parameters and the PHOTOSYSTEM II assumptions made when using these param- PHOTOCHEMISTRY .......... 91 eters to evaluate changes in photosynthetic Dark-Adapted State .............. 91 performance. Light-Adapted State .............. 95 RELATIONSHIP BETWEEN PHOTOSYSTEM II BACKGROUND OPERATING EFFICIENCY, Following the observation by Kautsky & LINEAR ELECTRON FLUX, Hirsch (55) that changes in fluorescence in- AND CO2 ASSIMILATION ..... 96 duced by illumination of dark-adapted leaves FACTORS THAT DETERMINE are qualitatively correlated with changes in PHOTOSYSTEM II CO assimilation, it became evident that un- OPERATING EFFICIENCY .... 98 2 der some circumstances fluorescence emis- Photochemical Quenching ........ 99 sions in photosynthetic organisms could be Nonphotochemical Quenching.... 101 correlated to their photosynthetic rates (54, IMAGING OF 56, 77). Butler (21) developed a simple model FLUORESCENCE .............. 104 for photosystem II (PSII) photochemistry in which photochemistry competes with the pro- cesses of fluorescence and heat loss for excita- tion energy in the pigment antenna of PSII INTRODUCTION (Figure 1). This model followed from the Heat loss: occurs The use of chlorophyll a fluorescence mea- proposal that electron transfer from the re- when excitation energy within surements to examine photosynthetic perfor- action center chlorophyll of PSII (P680) to pigments is lost as mance and stress in algae and plants is now the primary quinone acceptor of PSII (QA) heat; often termed widespread in physiological and ecophysio- quenches fluorescence (28), a process termed nonradiative decay logical studies. This has come about owing photochemical quenching. Increases in the or thermal to the development of a sound understanding rate of heat loss result in nonphotochemical deactivation of the relationships between fluorescence pa- quenching of fluorescence. The model pre- Excitation energy: rameters and photosynthetic electron trans- dicts that PSII fluorescence emission could by Miami University of Ohio on 09/23/10. For personal use only. energy within a pigment molecule port in vivo and the commercial availability of be used to monitor changes in photochem- after a photon is a range of affordable, easy to use portable flu- istry, provided that the rate constants for flu- Annu. Rev. Plant Biol. 2008.59:89-113. Downloaded from www.annualreviews.org absorbed and orimeters. Fluorescence can be a very power- orescence and heat loss do not change (21). generates an excited ful tool to study photosynthetic performance, However, it is now well established that large state of the molecule especially when coupled with other noninva- changes can occur in the rate constant for heat QA: primary sive measurements such as absorption spec- loss from the PSII antenna (61, 65). Con- quinone electron troscopy, gas analyses, and infrared thermom- sequently, to estimate PSII photochemistry acceptor of PSII etry. This review examines how some key from fluorescence, it is essential to determine Photochemical fluorescence parameters can be used to as- the fluorescence quenching that results from quenching: results from using excitation sess photosynthetic performance in vivo and both photochemical and nonphotochemical energy within to identify possible causes of changes in pho- processes. photosystem II tosynthesis and plant performance; it is aimed Separation of fluorescence quenching into (PSII) to drive at plant biologists who seek to use fluores- photochemical and nonphotochemical com- electron transport cence as a tool in their research. However, ponents was first achieved by the addition from P680 to Q A the underlying theoretical bases of fluores- of 3-(3,4-dichlorophenyl)-1,1-dimethylurea 90 Baker ANRV342-PP59-05 ARI 27 March 2008 1:28 (DCMU) to intact chloroplasts and Chlorella Light cells at points throughout the fluorescence induction curve (64, 66). DCMU inhibits electron transfer from QA to the secondary quinone acceptor of PSII (QB), which results in a rapid reduction of QA and an increase Photosystem II in fluorescence as photochemical quenching is prevented. A slower increase in fluores- cence follows, which is associated with the decay of nonphotochemical quenching. Un- Photochemistry fortunately, this DCMU technique is not suit- e– Q able for analyzing fluorescence quenching in P680 A leaves owing to the slow and uneven pen- etration of DCMU into leaf tissues. Also, Chlorophyll Heat the irreversibility of the DCMU inhibition fluorescence of electron transport makes the technique Figure 1 unsuitable for continuous measurements on Simple model of the possible fate of light energy absorbed by photosystem individual leaves. However, maximal QA re- II (PSII). Light energy absorbed by chlorophylls associated with PSII can duction in leaves in the light can be achieved be used to drive photochemistry in which an electron (e−) is transferred by rapidly exposing leaves to a very large in- from the reaction center chlorophyll, P680, to the primary quinone crease in light (17). This light-addition tech- acceptor of PSII, QA. Alternatively, absorbed light energy can be lost from nique is used to quantitatively determine the PSII as chlorophyll fluorescence or heat. The processes of photochemistry, chlorophyll fluorescence, and heat loss are in direct competition for fraction of fluorescence quenching that is excitation energy. If the rate of one process increases the rates of the other attributable to photochemical and nonphoto- two will decrease. chemical quenching processes (18). The de- lated to the quantum yield of CO assimilation velopment of fluorimeters that use weak mod- 2 by the leaf, φ (37), thus allowing, under ulated measuring beams in which phase and CO2 certain conditions, the application of fluores- Nonphotochemical frequency decoding are used to detect fluo- cence measurements to provide a rapid, non- quenching: occurs rescence yield changes enabled the routine, when there is an destructive probe of CO assimilation. A list nondestructive, quantitative determination of 2 increase in the rate at of the fluorescence parameters used in this re- photochemical and nonphotochemical pro- which excitation view, their definitions, and comments on their cesses in leaves by the application of a brief energy within physiological relevance are given in Table 1. photosystem II is lost (less than 1 s) saturating flash of light suffi- by Miami University of Ohio on 09/23/10. For personal use only. as heat ciently intense as to maximally reduce the QA PHOTOSYSTEM II Quantum yield pool in the sample (26, 102). The value of the (quantum Annu. Rev. Plant Biol. 2008.59:89-113. Downloaded from www.annualreviews.org modulated technique is that it provides a con- PHOTOCHEMISTRY efficiency) of a tinuous measure of the relative quantum yield process: number of Dark-Adapted State of fluorescence (101). This technique was used molecules undergoing the to demonstrate that the quantum yield of PSII When a leaf is kept in the dark, QA becomes process divided by photochemistry of a leaf at a given actinic maximally oxidized and the PSII reaction cen- the number of light intensity can be estimated from the mod- ters are referred to as being ‘open’, i.e., capa- photons absorbed by ulated fluorescence yield prior to the appli- ble of performing photochemical reduction of the system cation of the saturating flash and the maxi- QA.
Details
-
File Typepdf
-
Upload Time-
-
Content LanguagesEnglish
-
Upload UserAnonymous/Not logged-in
-
File Pages28 Page
-
File Size-