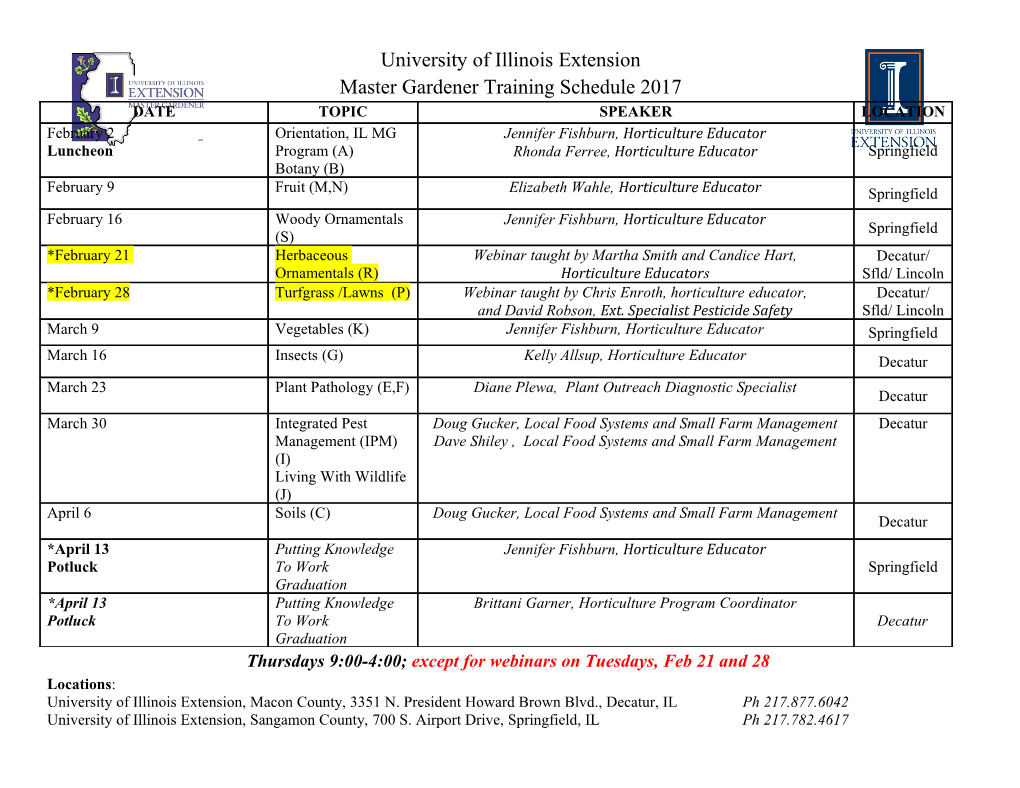
Principles of Semiconductor Devices . Principles of Semiconductor Devices B. Van Zeghbroeck . Principles of Semiconductor Devices ● Help ● Table of Contents ● Slideshow ● Movie http://ece-www.colorado.edu/~bart/book/ [2/28/2002 5:26:10 PM] Principles of Semiconductor Devices Principles of Semiconductor Devices ● Help ● Table of Contents ● Slideshow ● Movie http://ece-www.colorado.edu/~bart/book/book/title.htm [2/28/2002 5:26:12 PM] Principles of Semiconductor Devices Appendix: Appendix 1: List of Symbols Symbol Description MKS Units A Area m2 c Speed of light in vacuum m/s C Capacitance per unit area F/m2 CFB Flatband capacitance per unit area of a MOS structure F/m2 Cj Junction capacitance per unit area F/m2 Cox Oxide capacitance per unit area F/m2 Dn Electron diffusion constant m2/s Dp Hole diffusion constant m2/s E Energy Joule E Electric field V/m Ea Acceptor energy Joule Ec Conduction band energy of a semiconductor Joule Ed Donor energy Joule EF Fermi energy (thermal equilibrium) Joule Eg Energy bandgap of a semiconductor Joule Ei Intrinsic Fermi energy Joule Ev Valence band energy of a semiconductor Joule Evacuum Electron energy in vacumm Joule f(E) Distribution function (probability density function) Fn Quasi-Fermi energy of electrons Joule Fp Quasi-Fermi energy of holes Joule Density of states in the conduction band per unit energy and g (E) m-3J-1 c per unit volume Density of states in the valence band per unit energy and per g (E) m-3J-1 v unit volume Gn Electron generation rate m-3s-1 Gp Hole generation rate m-3s-1 h Plank's constant Js Reduced Plank's (= h /2π) Js I Current A J Current density A/m2 Jn Electron current density A/m2 Jp Hole current density A/m2 k Boltzmann's constant J/K l Mean free path m http://ece-www.colorado.edu/~bart/book/book/append/append1.htm (1 of 4) [2/28/2002 5:26:15 PM] Principles of Semiconductor Devices L Length m Ln Electron diffusion length m Lp Hole diffusion length m m Mass kg m0 Free electron mass kg * me Effective mass of electrons kg * mh Effective mass of holes kg n Electron density m-3 ni Intrinsic carrier density m-3 n(E) Electron density per unit energy and per unit volume m-3 n0 Electron density in thermal equilibrium m-3 ni Intrinsic carrier density m-3 N Doping density Na Acceptor doping density m-3 - -3 Na Ionized acceptor density m NB Base doping density m-3 Nc Effective density of states in the conduction band m-3 NC Collector doping density m-3 Nd Donor doping density m-3 + -3 Nd Ionized donor density m NE Emitter doping density m-3 Nv Effective density of states in the valence band m-3 p Hole density m-3 p(E) Hole density per unit energy m-3 p0 Hole density in thermal equilibrium m-3 pn Hole density in an n-type semiconductor m-3 q electronic charge C Q Charge C Charge density per unit area in the depletion layer of an MOS Q C/m2 d structure Charge density per unit area at threshold in the depletion layer Q C/m2 d,T of an MOS structure Qi Interface charge density per unit area C/m2 R Resistance Ohm Rn Electron recombination rate m-3s-1 Rp Hole recombination rate m-3s-1 t Thickness m tox Oxide thickness m T Temperature Kelvin Un Net recombination rate of electrons m-3s-1 Up Net recombination rate of holes m-3s-1 http://ece-www.colorado.edu/~bart/book/book/append/append1.htm (2 of 4) [2/28/2002 5:26:15 PM] Principles of Semiconductor Devices v Velocity m/s vth Thermal velocity m/s Va Applied voltage V VB Base voltage V VC Collector voltage V VD Drain voltage V VE Emitter voltage V VFB Flatband voltage V VG Gate voltage V Vt Thermal voltage V VT Threshold voltage of an MOS structure V w Depletion layer width m wB Base width m wC Collector width m wE Emitter width m wn Width of an n-type region m wp Width of a p-type region m x Position m xd Depletion layer width in an MOS structure m xd,T Depletion layer width in an MOS structure at threshold m xj Junction depth m xn Depletion layer width in an n-type semiconductor m xp Depletion layer width in a p-type semiconductor m a Transport factor b Current gain g Body effect parameter V1/2 gE Emitter efficiency d n Excess electron density m-3 d p Excess hole density m-3 DQn,B Excess electron charge density in the base C/m2 eox Dielectric constant of the oxide F/m es Dielectric constant of the semiconductor F/m mn Electron mobility m2/V-s mp Hole mobility m2/V-s Charge density per unit volume C/m3 r Resistivity Ωm rox Charge density per unit volume in the oxide C/m3 s Conductivity Ω−1m-1 tn Electron lifetime s http://ece-www.colorado.edu/~bart/book/book/append/append1.htm (3 of 4) [2/28/2002 5:26:15 PM] Principles of Semiconductor Devices tp Hole lifetime s f Potential V fB Barrier height V fF Bulk potential V fi Built-in potential of a p-n diode or Schottky diode V fs Potential at the semiconductor surface V FM Workfunction of the metal V Workfunction difference between the metal and the F V MS semiconductor FS Workfunction of the semiconductor V c Electron affinity of the semiconductor V http://ece-www.colorado.edu/~bart/book/book/append/append1.htm (4 of 4) [2/28/2002 5:26:15 PM] MOS Field-Effect-Transistors Chapter 7: MOS Field-Effect-Transistors 7.1. Introduction The n-type Metal-Oxide-Semiconductor Field-Effect-Transistor (nMOSFET) consists of a source and a drain, two highly conducting n-type semiconductor regions, which are isolated from the p-type substrate by reversed-biased p-n diodes. A metal or poly-crystalline gate covers the region between source and drain. The gate is separated from the semiconductor by the gate oxide. The basic structure of an n-type MOSFET and the corresponding circuit symbol are shown in Figure 7.1.1. Figure 7.1.1 : Cross-section and circuit symbol of an n-type Metal-Oxide-Semiconductor-Field-Effect-Transistor (MOSFET) As can be seen on the figure the source and drain regions are identical. It is the applied voltages, which determine which n- type region provides the electrons and becomes the source, while the other n-type region collects the electrons and becomes the drain. The voltages applied to the drain and gate electrode as well as to the substrate by means of a back contact are referred to the source potential, as also indicated Figure 7.1.1. A conceptually similar structure was proposed and patented independently by Lilienfeld and Heil in 1930, but was not successfully demonstrated until 1960. The main technological problem was the control and reduction of the surface states at the interface between the oxide and the semiconductor. Initially it was only possible to deplete an existing n-type channel by applying a negative voltage to the gate. Such devices have a conducting channel between source and drain even when no gate voltage is applied and are called "depletion-mode" devices. A reduction of the surface states enabled the fabrication of devices, which do not have a conducting channel unless a positive voltage is applied. Such devices are referred to as "enhancement-mode" devices. The electrons at the oxide- semiconductor interface are concentrated in a thin (~10 nm thick) "inversion" layer. By now, most MOSFETs are "enhancement-mode" devices. While a minimum requirement for amplification of electrical signals is power gain, one finds that a device with both voltage and current gain is a highly desirable circuit element. The MOSFET provides current and voltage gain yielding an output current into an external load which exceeds the input current and an output voltage across that external load which exceeds the input voltage. http://ece-www.colorado.edu/~bart/book/book/chapter7/ch7_1.htm (1 of 2) [2/28/2002 5:26:16 PM] MOS Field-Effect-Transistors The current gain capability of a Field-Effect-Transistor (FET) is easily explained by the fact that no gate current is required to maintain the inversion layer and the resulting current between drain and source. The device has therefore an infinite current gain in DC. The current gain is inversely proportional to the signal frequency, reaching unity current gain at the transit frequency. The voltage gain of the MOSFET is caused by the current saturation at higher drain-source voltages, so that a small drain- current variation can cause a large drain voltage variation. http://ece-www.colorado.edu/~bart/book/book/chapter7/ch7_1.htm (2 of 2) [2/28/2002 5:26:16 PM] MOS Capacitors Chapter 6: MOS Capacitors 6.1. Introduction The primary reason to study the Metal-Oxide-Silicon (MOS) capacitor is to understand the principle of operation as well as the detailed analysis of the Metal-Oxide-Silicon Field Effect Transistor (MOSFET). In this chapter, we introduce the MOS structure and its four different modes of operation, namely accumulation, flatband, depletion and inversion. We then consider the flatband voltage in more detail and present the MOS analysis based on the full depletion approximation. Finally, we analyze and discuss the MOS capacitance.
Details
-
File Typepdf
-
Upload Time-
-
Content LanguagesEnglish
-
Upload UserAnonymous/Not logged-in
-
File Pages515 Page
-
File Size-