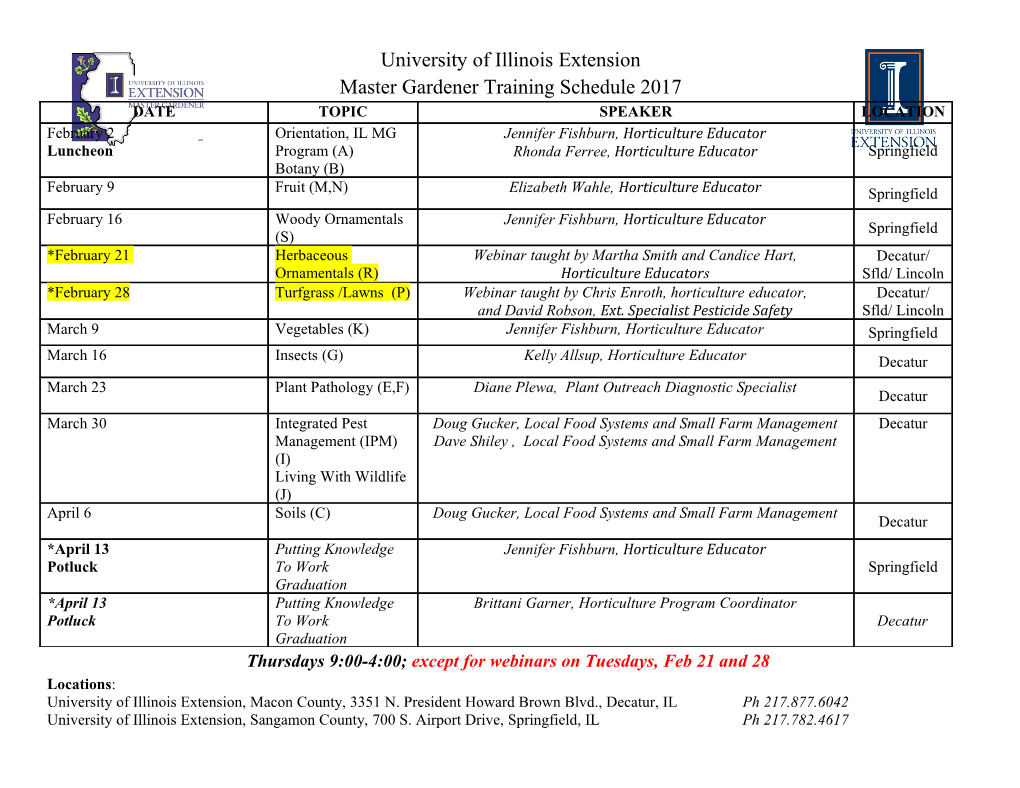
Physiol Rev 87: 659–797, 2007; doi:10.1152/physrev.00043.2006. Physiology and Pathophysiology of Purinergic Neurotransmission GEOFFREY BURNSTOCK Autonomic Neuroscience Centre, Royal Free and University College Medical School, London, United Kingdom I. Introduction 660 II. Background 660 A. Autonomic neuromuscular transmission 661 B. Autonomic ganglia 662 C. Central nervous system 662 Downloaded from D. Purinergic receptor subtypes 664 E. ATP storage, release, and breakdown 667 F. Plasticity of purinergic signaling 668 III. ATP as a Cotransmitter 670 A. Sympathetic nerves 670 B. Parasympathetic nerves 672 C. Sensory-motor nerves 672 physrev.physiology.org D. Intrinsic nerves in the gut and heart 672 E. Peripheral motor nerves 673 F. Nerves in the brain and spinal cord 673 IV. Neurotransmission and Neuromodulation in Autonomic Ganglia 674 A. Sympathetic ganglia 674 B. Adrenal chromaffin cells 675 C. Parasympathetic ganglia 676 D. Enteric ganglia 676 V. Sensory Neurons 679 on April 19, 2007 A. Dorsal root ganglia 680 B. Nodose ganglia 681 C. Trigeminal ganglia 681 D. Petrosal ganglia 682 E. Retinal ganglia 682 F. Sensory nerve fibers and terminals 682 VI. Neurotransmission and Neuromodulation in the Central Nervous System 685 A. Cortex 686 B. Hippocampus 687 C. Cerebellum 688 D. Basal ganglia 689 E. Midbrain 690 F. Thalamus 690 G. Habenula 690 H. Behavioral studies 690 VII. Central Control of Autonomic Function 693 A. Ventrolateral medulla 693 B. Trigeminal mesencephalic nucleus 693 C. Area postrema 694 D. Locus coeruleus 694 E. Nucleus tractus solitarius 694 F. Motor and sensory nuclei 695 G. Hypothalamus 696 H. Spinal cord 697 VIII. Neuron-Glia Interactions 698 A. P1 and P2 receptors on glial cells 698 B. Neuron-astrocyte interactions 700 C. Interactions of axons with Schwann cells and oligodendrocytes 702 D. Interactions between neurons and microglia 703 www.prv.org 0031-9333/07 $18.00 Copyright © 2007 the American Physiological Society 659 660 GEOFFREY BURNSTOCK IX. Purinergic Neuroeffector Transmission 704 A. Exocrine glands 704 B. Endocrine and neuroendocrine cells 705 C. Endothelial cells 707 D. Secretory epithelial cells in visceral organs 707 E. Immune cells 709 F. Bone cells, joints, and keratinocytes 710 G. Choroid plexus 711 H. Interstitial cells of Cajal 711 I. Sensory epithelia 711 X. Ontogeny and Phylogeny of Purinergic Neurotransmission 712 A. Pre- and postnatal development and aging 712 B. Purinergic neurotransmission in invertebrates and lower vertebrates 722 XI. Neuropathology 729 A. Peripheral nervous system 729 B. Central nervous system 740 XII. Concluding Comments and Future Directions 749 Burnstock G. Physiology and Pathophysiology of Purinergic Neurotransmission. Physiol Rev 87: 659–797, 2007; Downloaded from doi:10.1152/physrev.00043.2006.—This review is focused on purinergic neurotransmission, i.e., ATP released from nerves as a transmitter or cotransmitter to act as an extracellular signaling molecule on both pre- and postjunctional membranes at neuroeffector junctions and synapses, as well as acting as a trophic factor during development and regeneration. Emphasis is placed on the physiology and pathophysiology of ATP, but extracellular roles of its breakdown product, adenosine, are also considered because of their intimate inter- actions. The early history of the involvement of ATP in autonomic and skeletal neuromuscular transmission and in activities in the central nervous system and ganglia is reviewed. Brief background information is given about physrev.physiology.org the identification of receptor subtypes for purines and pyrimidines and about ATP storage, release, and ectoenzymatic breakdown. Evidence that ATP is a cotransmitter in most, if not all, peripheral and central neurons is presented, as well as full accounts of neurotransmission and neuromodulation in autonomic and sensory ganglia and in the brain and spinal cord. There is coverage of neuron-glia interactions and of purinergic neuroeffector transmission to nonmuscular cells. To establish the primitive and widespread nature of puriner- gic neurotransmission, both the ontogeny and phylogeny of purinergic signaling are considered. Finally, the pathophysiology of purinergic neurotransmission in both peripheral and central nervous systems is reviewed, and speculations are made about future developments. on April 19, 2007 I. INTRODUCTION II. BACKGROUND It is nearly 35 years ago that I published a paper Extracellular actions of purine nucleotides and entitled “Purinergic Nerves” in Pharmacological Re- nucleosides were first described in a seminal paper by views (241). It was a new hypothesis backed by some Drury and Szent-Gyo¨ rgyi in 1929 (481) in the cardiovas- good evidence for ATP as a neurotransmitter in nonad- cular system, and later in the uterus (451) and intestine (642). Studies of the effects of purines on the nervous renergic, noncholinergic nerves supplying the gut and system followed the early emphasis on their cardiovascu- bladder and included every hint that I could find to lar actions. There was early recognition for a physiologi- support the possible involvement of purinergic signal- cal role for ATP at the skeletal neuromuscular junction. ing in different parts of the nervous system. However, it Buchthal and Folkow (226) injected ATP into the sciatic was regarded with skepticism by a large number of artery supplying the gastrocnemius muscle of the frog and people over the next 20 years. So this current review of reported tetanus-like contractions; they also observed purinergic neurotransmission, with a huge and rapidly that the sensitivity of the preparation to ACh was greatly growing body of evidence for purinergic involvement in increased by previous application of ATP (227, 228). Parts both physiological and pathophysiological neural of the spinal cord were shown to be sensitive to ATP mechanisms, is for me, a rather emotional vindication (225). Emmelin and Feldberg (518) found complex effects of my life’s work. It is a comprehensive account, and initiated by intravenous injection of ATP into cats affect- therefore, I hope I will be forgiven for its length and for ing peripheral, reflex, and central mechanisms. Injection the long list of papers, although reference to review of ATP into the lateral ventricle of the cat produced articles is made wherever possible to cover some of the muscular weakness, ataxia, and a tendency of the animal earlier literature. to sleep (538). The application of adenosine or ATP to Physiol Rev • VOL 87 • APRIL 2007 • www.prv.org PURINERGIC NEUROTRANSMISSION 661 various regions of the brain produced biochemical or electrophysiological changes (74, 610, 1563). ATP and related nucleotides were shown to have anti-anesthetic actions (981). The first hint that ATP might be a neuro- transmitter in the peripheral nervous system (PNS) arose when it was proposed that ATP released from sensory nerves during antidromic nerve stimulation of the great auricular nerve caused vasodilatation in the rabbit ear artery (755, 756). The purinergic nerve hypothesis, with ATP as the transmitter responsible for nonadrenergic, noncholinergic (NANC) transmission to the smooth mus- cle of the gut and bladder, was proposed by Burnstock in 1972 (241). A brief historical review about the develop- ment of the concept of ATP as a neurotransmitter has been published recently (277). A. Autonomic Neuromuscular Transmission Downloaded from There was early recognition of atropine-resistant re- sponses of the gastrointestinal tract to parasympathetic nerve stimulation (999, 1138, 1321). However, it was not until the early 1960s that autonomic transmission other than adrenergic and cholinergic was established. In 1963, physrev.physiology.org electrical activity was recorded in the guinea pig taenia coli using the sucrose-gap technique, and after stimula- tion of the intramural nerves in the presence of adrenergic and cholinergic blocking agents, an inhibitory hyperpolar- izing potential was observed (282, 283). The hyperpolar- FIG. 1. Early experiments. A: sucrose gap recording of membrane izing responses were blocked by tetrodotoxin (TTX), a potential changes in smooth muscle of guinea pig taenia coli in the presence of atropine (0.3 M) and guanethidine (4 M). Transmural field neurotoxin that prevents the action potential in nerves stimulation (0.5 ms, 0.033 Hz, 8 V) evoked transient hyperpolarizations, on April 19, 2007 without affecting the excitability of smooth muscle cells which were followed by rebound depolarizations. Tetrodotoxin (TTX, 3 (233; Fig. 1A), indicating their neurogenic nature and M) added to the superfusing Kreb’s solution (applied at arrow) rapidly abolished the responses to transmural field stimulation, indicating that establishing them as inhibitory junction potentials (IJPs) they were inhibitory junction potentials in response to stimulation of in response to NANC nerves. This work was extended by an nonadrenergic noncholinergic (NANC) inhibitory nerves. [From Burn- analysis of the mechanical responses to NANC nerve stim- stock (249), with kind permission of Blackwell Publishing.] B: mechan- ical responses of the guinea pig taenia coli to intramural nerve stimula- ulation of the taenia coli (284). NANC mechanical responses tion (NS: 1 Hz, 0.5-ms pulse duration, for 10 s at supramaximal voltage) were also observed by Martinson and Muren in the cat and ATP (2 x 10Ϫ6 M). The responses consist of a relaxation followed by Ϫ stomach upon stimulation of the vagus nerve (1112), and a “rebound contraction.” Atropine
Details
-
File Typepdf
-
Upload Time-
-
Content LanguagesEnglish
-
Upload UserAnonymous/Not logged-in
-
File Pages139 Page
-
File Size-