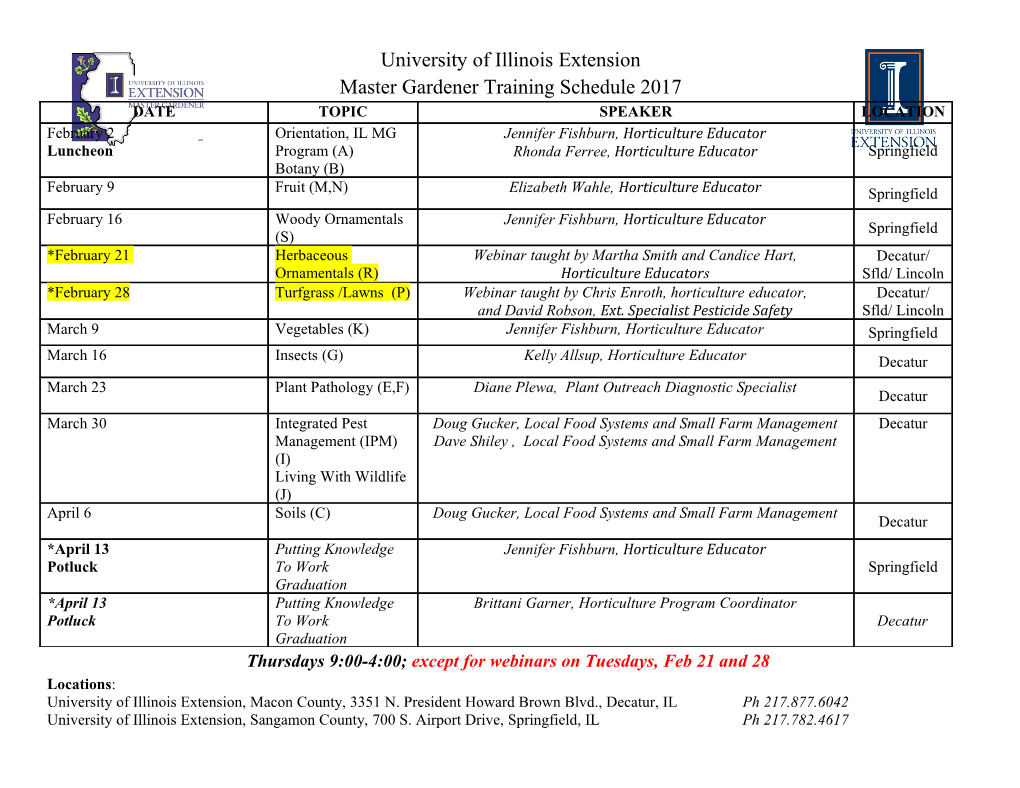
Numerical Integration MAT 2310 Computational Mathematics Wm C Bauldry November, 2011 ICM 1 Contents 1. Numerical Integration 5. Gaussian Quadrature 2. Left- and Right Endpoint, 6. Gauss-Kronrod Quadrature and Midpoint Sums 7. Test Integrals 3. Trapezoid Sums 8. Exercises 4. Simpson’s Rule 9. Links and Others Today ICM 2 Numerical Integration What is Numerical Integration? Numerical integration or (numerical) 2.5 quadrature is the calculation of a definite 2 1.5 integral using numerical formulas, not the 1 fundamental theorem. The Greeks stud- 0.5 ied quadrature: given a figure, construct a -1 -0.5 0 0.5 1 1.5 2 2.5 3 3.5 4 4.5 -0.5 square that has the same area. The two -1 most famous are Hippocrates of Chios’ Quadrature of the Lune (c. 450 BC) and Archimedes’ Quadrature of the Parabola (c. 250 BC). Archimedes used the method of exhaustion — a precursor of calculus — invented by Eudoxus. Squaring the circle is one of the classical problems of constructing a square with the area of a given circle – it was shown impossible by Lindemann’s theorem (1882). ICM 3 Methods of Elementary Calculus Rectangle Methods Left endpoint sum Midpoint sum Right endpoint sum n n n X X X An ≈ f(xk−1) ∆xk An ≈ f(mk) ∆xk An ≈ f(xk) ∆xk k=1 k=1 k=1 1 mk = 2 (xk−1 + xk) (b−a)2 1 (b−a)3 1 (b−a)2 1 "n ≤ · M1 · "n ≤ · M2 · "n ≤ · M1 · 2 n 24 n2 2 n where M = max f (i)(x) R πf dx = 2 ≈ [1:984; 2:008; 1:984] i 0 n=10 ICM 4 Trapezoid Sums Trapezoid Sums Instead of the degree 0 rectangle approximations to the function, use a linear degree 1 approximation. The area of the trapezoid is given by 1 AT = 2 [f(xk−1) + f(xk)] ∆xk This gives an approximation for the integral Z b n X 1 f(x) dx ≈ 2 [f(xk−1) + f(xk)] ∆xk a k=1 [Midpoint: measure height at average x v. trapezoid: average the height measures] The formula is often written as " n−1 ! # X ∆xk T ≈ f(x ) + 2 f(x ) + f(x ) n 0 k n 2 k=1 Error for the trapezoid rule is (b − a)3 1 " ≤ · M · n 12 2 n2 ICM 5 Sample Trapezoid Example 1 1 1 Let f(x) = sin(x) + 2 sin(2x) − 4 sin(4x) + 16 sin(8x) over [0; π]. With an equipartition, ∆x = π=10 ≈ 0:314 Then " 10 ! # X ∆x T = f(0)+ 2 f( k π) +f(π) 10 9 2 k=1 which gives T10 = 1:984 with absolute error of 0:016. ICM 6 Simpson’s Rule Simpson’s Rule We now move to a degree 2 approximation. The easiest way to have 3 data pts is to take the panels in pairs: instead of rectangle base [xi; xi+1], use [xi; xi+1; xi+2]. So we require an even number of panels. The area under the parabola is 1 h i AS = 3 f(xi) + 4f(xi+1) + f(xi+2) ∆x This gives a 2n-panel approximation for the integral " n # Z b X ∆x f(x) dx ≈ f(x ) + 4f(x ) + f(x ) 2k−2 2k−1 2k 3 a k=1 often written as ∆x S = [f(x ) + 4f(x ) + 2f(x ) + 4f(x ) + ··· + 4(f(x ) + f(x )] 2n 0 1 2 3 2n−1 2n 3 The error is bounded by (b − a)5 1 " ≤ · M · n 180 4 n4 ICM 7 Sample Simpson Example 1 1 1 Let f(x) = sin(x) + 2 sin(2x) − 4 sin(4x) + 16 sin(8x) over [0; π]. With a 10 panel equipartition, ∆x = π=10 ≈ 0:314 Then, with yi = f(xi), 1 S10 = 3 [y0 +4y1 +2y2 +···+4y9 +y10]∆x which gives S10 = 2:000 with absolute error of 6:78 · 10−6. ICM 8 A Maple Comparison Approximating a Difficult Integral Z 2 10−4 Consider dx. The integrand has a sharp peak at π=2. 1 2 −8 1 (x − 2 π) + 10 The exact value of the integral (FToC) is arctan(−10000 + 5000 π) + arctan(20000 − 5000 π) ≈ 3:14118447 Maple gives n = 50 n = 500 n = 5000 2 left 0:04972053 2 left 0:5393463 2 left 3:424973 6 right 0:04972537 6 right 0:5393457 6 right 3:424957 6 7 6 7 6 7 6 midpoint 3:09300007 6 midpoint 4:1670607 6 midpoint 2:880897 6 7 6 7 6 7 4trapezoid 0:04972285 4trapezoid 0:5393425 4trapezoid 3:424985 Simpson 2:0785900 Simpson 2:957850 Simpson 3:06226 To achieve relative error below 1% requires n ≥ 8000. ICM 9 Þe Charte Ob Anfeald Gang Quadrature “Height” Error Bound (b−a)2 1 Left end point f(xi) 2 · M1 · n (b−a)2 1 Right end point f(xi+1) 2 · M1 · n 3 f 1 (x + x ) (b−a) · M · 1 Midpoint 2 i i+1 24 2 n2 3 1 (f(x ) + f(x )) (b−a) · M · 1 Trapezoid Rule 2 i i+1 12 2 n2 1 (b−a)5 1 Simpson’s Rule 3 (f(xi) + 4f(xi+1) + f(xi+2)) 180 · M4 · n4 (i) where Mi ≥ max f (x) . ICM 10 Gaussian Quadrature Johann Carl Friedrich Gauss About 1815, while Gauss was finishing constructing astronomical observat- ory, he wrote a paper1 on approximating integrals. Gauss’s technique was studied and extended by Christoffel in 1858. There are several ways to develop his method. We’ll use the easiest . In Search of Improvements Write the rules we’ve seen as sums: 1 1 1 Left endpt: Ln= n f(x0) + n f(x1) + ··· + n f(xn−1) 1 1 1 Right endpt: Rn= n f(x1) + n f(x2) + ··· + n f(xn) 1 1 1 Midpoint:Mn= n f(xm1 ) + n f(xm2 ) + ··· + n f(xmn ) 1 1 1 1 Trapezoid: Tn= 2n f(x0) + n f(x1) + ··· + n f(xn−1) + 2n f(xn) 1 4 2 4 1 Simpson’s: Sn= 3n f(x0) + 3n f(x1) + 3n f(x2) + ··· + 3n f(xn−1) + 3n f(xn) 1 “Methodus nova integralium valores per approximationem inveniendi,” Werke, 3, K. Ges. Wissenschaft. Göttingen (1886) pp. 163–196. ICM 11 Patterns Observe 1. Each of the formulas has the same form An = w1 · f(x1) + w2 · f(x2) + ··· + wn · f(xn) with different sets of weights wi and different sets of nodes xi. 2. Any closed interval can be mapped to and from [−1; 1], so we R 1 can focus only on −1 f(x) dx. 3. Gauss posed the question: Is there a “best choice” of weights fwig and nodes fxig? 4. The answer depends on what “best” means. 5. Since we have 2n ‘unknowns’ wi and xi, let’s look for a set that integrates a 2n − 1 degree polynomial exactly. ICM 12 Sampling 3 Example (Third Degree) Set n = 3. Determine the choice of wi and of xi so that Z 1 3 p X p x dx = wk · (xk) −1 k=1 exactly for p = 0; 1;:::; 5 = 2 · 3 − 1. The range for p gives us six equations: 8 9 > w1 + w2 + w3 = 2 > > > > > > w x + w x + w x = 0 > > 1 1 2 2 3 3 > 8 p p > > 15 15 > 2 2 2 2 > > x1 = − 5 ; x2 = 0; x3 = 5 < w1x1 + w2x2 + w3x3 = = < 3 =) > w x3 + w x3 + w x3 = 0 > > > 1 1 2 2 3 3 > > w = 5 ; w = 8 ; w = 5 > > : 1 9 2 9 3 9 > 4 4 4 2 > > w1x1 + w2x2 + w3x3 = 5 > > > > 5 5 5 > : w1x1 + w2x2 + w3x3 = 0 ; p p 5 15 8 5 15 Our Gaussian quadrature is G3(f) = 9 f − 5 + 9 f(0) + 9 f 5 ICM 13 Testing Gauss Random Polynomials Generate and test a random 5th degree polynomial. p := unapply(sort(randpoly(x, degree = 5), x), x) x ! −7x5 + 22x4 − 55x3 − 94x2 + 87x − 56 G3 := 5/9*p(-sqrt(15)/5) + 8/9*p(0) + 5/9*p(sqrt(15)/5) 2488 − 15 Int(p(x), x = -1..1) = int(p(x), x = -1..1) Z 1 2488 p(x) dx = − 15 −1 Generate and test a random 7th degree polynomial. q := unapply(sort(randpoly(x, degree = 7), x), x) x ! 97x7 − 73x6 − 4x5 − 83x3 − 10x − 62 int(q(x),x= -1..1)= 5/9*q(-sqrt(15)/5)+8/9*q(0)+5/9*q(sqrt(15)/5) 722 2662 7 = 25 ICM 14 Gaussian Properties Theorem Z 1 Let f have 2n continuous derivatives. Then for "n = Gn − f(x)dx , 2 −1 " ≤ · M 2n n (2n + 1)! (2n) where M2n ≥ max f (x) . Values of Gaussian Weights and Nodes There are numerous sources online, e.g.,: 1. The classic Abramowitz and Stegun Handbook (see the entire book) 2. Holoborodko or Kamermans Or calculate the values directly: 1 dn 2 n Set Pn(x) = 2n n! · dxn x − 1 (the Legendre polynomials). Then n 2 fxigi=1 = fzeros of Png and wi = 2 0 2 (1 − xi )[Pn(xi)] ICM 15 Aleksandr Kronrod Kronrod’s Idea (1964) One difficulty in Gaussian quadrature is that increasing the number of nodes requires recomputing all the values of • nodes • weights • function evaluations Kronrod2 discovered he could interlace n + 1 new nodes with n original Gaussian nodes and have a rule of order 3n + 1. A 2n + 1 node Gaussian quadrature would have order 4n + 1, but with significant extra computation for an increase of only n in order over Kronrod’s method.
Details
-
File Typepdf
-
Upload Time-
-
Content LanguagesEnglish
-
Upload UserAnonymous/Not logged-in
-
File Pages23 Page
-
File Size-