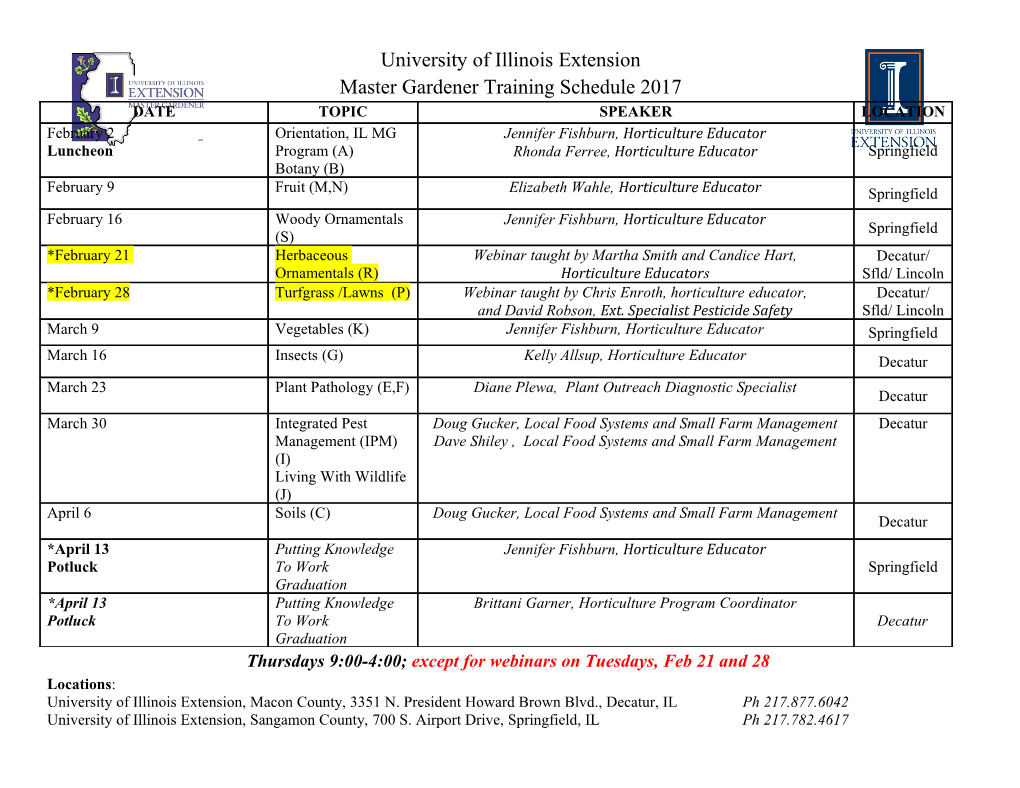
Geophys. J. Int. (2005) 163, 760–774 doi: 10.1111/j.1365-246X.2005.02725.x Mantle tectonics beneath New Zealand inferred from SKS splitting and petrophysics Mathieu Duclos,1,2 Martha K. Savage,1 Andr´ea Tommasi2 and Ken R. Gledhill3 1Institute of Geophysics, School of Earth Sciences, PO Box 600, Victoria University of Wellington, Wellington 6001, New Zealand. E-mail: [email protected] 2Laboratoire de Tectonophysique, ISTEEM, CNRS, Universit´e Montpellier 2, France 3Institute of Geological and Nuclear Sciences, Lower Hutt, New Zealand Accepted 2005 June 27. Received 2005 February 21; in original form 2004 October 31 SUMMARY We constrain the extent of lithospheric and asthenospheric deformation beneath New Zealand by coupling measurements of shear wave splitting of teleseismic waves and petrophysical analysis of mantle xenoliths. SKS splitting for the central South Island and the eastern part of the North Island confirms earlier observations. The fast S-wave polarization directions are roughly NE/SW, which is parallel to the Alpine Fault in the South Island and to the strike of the Hikurangi subduction zone in the North Island. This suggests that flow parallel to the plate boundary extends up to 160 km away from the boundary. Departures from this pattern are restricted to the southernmost station and the two northernmost stations and indicate changes in the mantle flow or a more complex anisotropic pattern, such as a dipping axis of symmetry or heterogeneous anisotropy. Analysis of mantle xenoliths from the Raglan (North Island), Dunedin (South Island) and Chatham Island regions allows us to constrain the lithospheric contribution to the observed seismic anisotropy. The intrinsic S-wave anisotropy is 1.5 per cent higher for the South Island samples (∼5.0 per cent versus ∼3.5 per cent for the North Island), indicating a strong lithospheric deformation that could explain the major part of the observed splitting. In the North Island, the intrinsic lithospheric anisotropy is too low to explain the SKS splitting times. This indicates that asthenospheric deformation probably plays a major role there. For the Chatham Island, the anisotropy pattern remains unresolved as the xenolith suggests strong lithospheric anisotropy but no present splitting is measured. Key words: mantle tectonics, New Zealand, petrophysics, seismic anisotropy, shear wave splitting. GJI Tectonics and geodynamics thenospheric deformations. New Zealand is the perfect place for INTRODUCTION the study of the mantle flow beneath an oblique convergent plate When a shear wave enters an anisotropic medium, it is split in boundary given the good knowledge of the relative movements of two perpendicular directions corresponding to the fast and slow the plates creating the boundary and the large number of geophysi- polarization directions of the anisotropic body for its particular cal studies conducted there recently. Moreover, lithospheric mantle path (Crampin 1985). Using a three-component seismometer, one xenoliths can be retrieved in the North and South Islands as well can record these two split phases and evaluate their time differ- as the Chatham Islands. The petrophysical study of these xenoliths ence and the direction of polarization of the fast component of the allows constraints on the lithospheric contribution to the observed S wave (termed ‘fast direction’ hereafter). Flow in the shallow man- splitting. tle (at depths <200–250 km) leads to alignment of the [100]-axis of olivine parallel or subparallel to the shearing direction (Nico- GEODYNAMIC CONTEXT las & Christensen 1987) and the fast direction measured with tele- OF THE STUDY seismic shear wave splitting indicates the flow direction under the recording seismic station. Due to the lack of vertical resolution of The two main islands of New Zealand are situated on a segment this technique, two end-member hypotheses can be considered; one of the obliquely convergent boundary between the Australian and links the anisotropy to the deformation of the lithosphere, the other Pacific plates (Fig. 1). The relative movement of these plates varies links the anisotropy to an asthenospheric flow. The most probable from 47 mm yr−1 along the northern end of the Hikurangi margin hypothesis is that the SKS wave records both lithospheric and as- down to 38 mm yr−1 along the Alpine Fault (DeMets et al. 1994). 760 C 2005 RAS Mantle tectonics beneath New Zealand 761 Figure 1. Major structures and geodynamic regions of New Zealand: the Hikurangi subduction zone and the associated Central volcanic region (CVR), the Marlborough Fault System (MFS), the Alpine Fault and the Puysegur subduction zone. The stars represent the localities from which our mantle xenolithswere obtained. From North to South, we can divide New Zealand into four major gur subduction is not well constrained. The South Island of New geodynamic regions (Fig. 1). The North Island is characterized by Zealand is thus a transition between two subductions of opposite the active west-dipping Hikurangi subduction zone and the Central dip, the Hikurangi and Puysegur subduction zones, through a set of volcanic region that is undergoing active volcanism through backarc dextral transpressive faults: the MFS and the Alpine Fault. Here, we extension (Adams & Hatherton 1973). In the South Island, the major focus on three of the major regions, the two subduction zones and tectonic feature is the Alpine Fault. This dextral transform boundary the Alpine Fault regions. has been active for ∼45 Ma (Cooper & Norris 1994; Walcott 1998). About 6.4 Ma ago, a change in direction of the plate motions led to a PETROPHYSICS DATA stronger compressional motion, transforming the Alpine Fault into a transpressive system with a vertical component of movement three In order to constrain the lithospheric contribution to the observed times smaller than the horizontal one (Walcott 1998). This com- splitting, we conducted a joint petrological and petrophysical anal- pression might have started even earlier, possibly 20 Ma ago but ysis of eight mantle xenoliths brought up to the surface by vari- with less vertical movement, as inferred from a recent palaeogeog- ous phases of intraplate basaltic volcanism. Three spinel-lherzolites raphy reconstruction taking into account a microplate southwest of (9225, 9231 and 5548) were brought to the surface by intraplate New Zealand, the Macquarie Plate (Cande & Stock 2004). Between basaltic volcanism active from 2.8 Ma to 1.7 Ma in the Raglan area these two systems, the Marlborough Fault System (MFS) and its in the North Island (Kear 1994). Three spinel-lherzolites and one extension in the lower North Island, the Wellington region, are a spinel-harzburgite come from the Dunedin area in the South Island transition zone composed of a set of dextral transpressive faults. In (D104A, D105C, D106C, D120B). In that area, the volcanic ac- the southernmost part of the South Island, the Fiordland region is tivity, consisting mainly of mantle derived alkali basalts (Bishop an anomalously elevated zone (Oliver & Coggon 1979), linked to &Turnbull 1996), began at 21 Ma, ending around 9 Ma (Coombs the steeply northeast-dipping Puysegur subduction zone (Eberhart- et al. 1986; Coombs 1987). The last sample, the spinel-lherzolite Phillips & Reyners 2001; Smith 1971). The transition between the NZ56CH comes from a 60 Ma old volcanic flow from the Chatham Alpine Fault, which disappears in the Tasman Sea, and the Puyse- Islands (Hoke et al. 2000). C 2005 RAS, GJI, 163, 760–774 762 M. Duclos et al. Figure 2. Olivine and enstatite measured lattice preferred orientations. Leftmost three plots are lower hemisphere equal area projection for olivine LPO, contours at 0.5 multiple of a uniform distribution interval. Rightmost three plots are lower hemisphere projection for enstatite LPO, dotted due to low number of measurements. A horizontal line marks an observed foliation plane where it is present. Other samples’ LPOs are rotated to align the [100] axes with the X direction. The number of crystals measured is shown in the upper left corner. C 2005 RAS, GJI, 163, 760–774 Mantle tectonics beneath New Zealand 763 Table 1. Modal composition, main mineralogical characteristics and average modelled temperature of the xenoliths. Mg# = 100 × (Mg/(Mg+Fe)) with Mg and Fe the atomic percentage of magnesium and iron respectively. Cr# = 100 × (Cr/(Cr+Al)) with Cr and Al the atomic percentage of chromium and aluminium, respectively. Sample Modal composition (%) Main mineralogical characteristics Avg temperature Olivine OrthoPx ClinoPx Spinel Mg# Olivine Mg# OrthoPx Mg# Spinel Cr# Spinel Al Content (%) (◦C) 5548 75 8.5 6.5 3 89.8 89.9 73.6 14.7 5.13 1005 9225 54 23 13.5 4 89.9 90.1 72.7 15.7 5.62 1030 9231 63 19 8 2 90.8 91.1 66.2 38.5 4.37 1160 D104A 56 20 18 3 89.7 90.8 79.5 10 4.91 950 D105C 65 26 6 2 91.5 92.5 79.1 27.1 3 935 D106C 81 12 3 1 90.7 92.3 58.9 61 2.2 1050 D120B 64 18 13 2 90.2 90.8 78.5 12.7 5.4 1045 NZ56CH 67 24 5 1 92.1 92.1 67 55.4 1.6 670 Analysis of the microstructure by optical and electron microscopy samples), from secondary activation of low-temperature [001] slip, and measurement of strain-induced crystal preferred orientations by or from dynamic recrystallization (Tommasi et al. 2000). indexation of electron backscattered diffraction (ESBD) patterns are We calculated the average seismic properties for the North and used to unravel the deformation history. In order to characterize the South Islands by adding the olivine and pyroxene LPOs of all the lithospheric mantle composition at each site, modal and mineral samples from each area (Fig. 3). The North Island average sam- compositions (Table 1) are determined through analysis of electron ple presents a 3.45 ± 0.4 per cent maximum S-wave polarization backscattered images of thin sections and microprobe analysis, re- anisotropy for a wave travelling in the foliation plane normal to the spectively.
Details
-
File Typepdf
-
Upload Time-
-
Content LanguagesEnglish
-
Upload UserAnonymous/Not logged-in
-
File Pages15 Page
-
File Size-