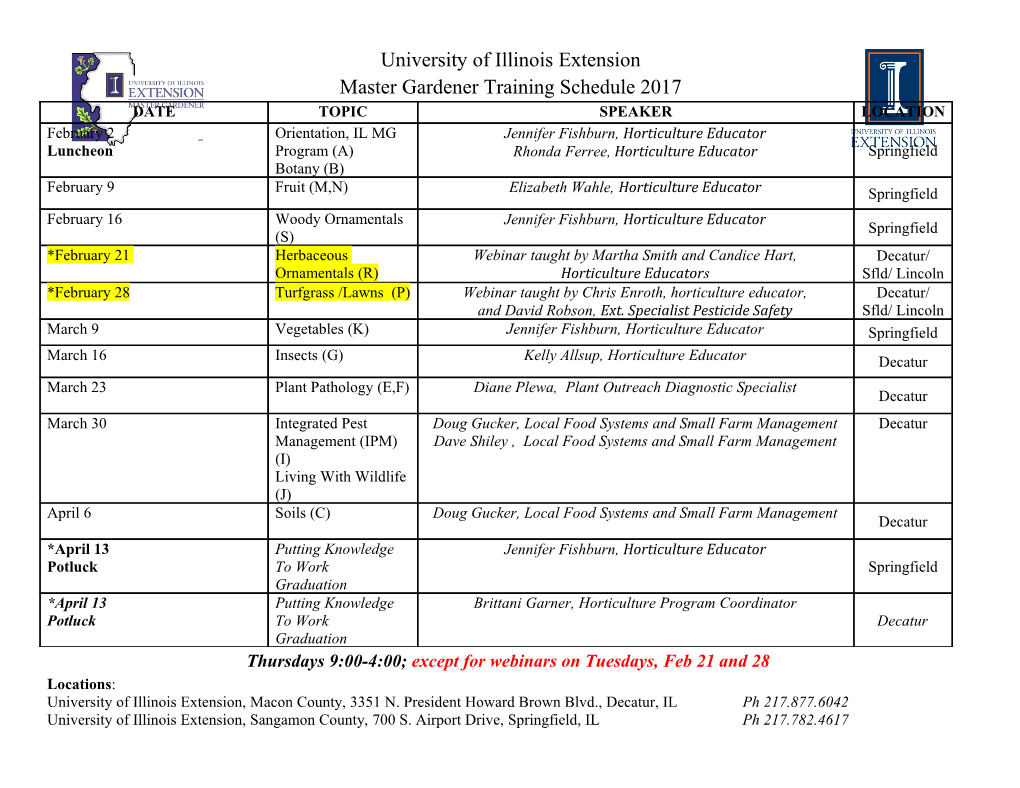
ANALYSIS TOOLS WITH APPLICATIONS 533 29. Unbounded operators and quadratic forms 29.1. Unbounded operator basics. Definition 29.1. If X and Y are Banach spaces and D is a subspace of X,thena linear transformation T from D into Y is called a linear transformation (or operator) from X to Y with domain D. We will sometimes wr If D is dense in X, T is said to be densely defined. Notation 29.2. If S and T are operators from X to Y with domains D(S)and D(T )andifD(S) D(T )andSx = Tx for x D(S), then we say T is an extension of S and write⊂ S T. ∈ ⊂ We note that X Y is a Banach space in the norm × x, y = x 2 + y 2. kh ik k k k k If H and K are Hilbert spaces, then Hp K and K H become Hilbert spaces by defining × × ( x, y , x0,y0 )H K := (x, x0)H +(y,y0)K h i h i × and ( y, x , y0,x0 )K H := (x, x0)H +(y,y0)K . h i h i × Definition 29.3. If T is an operator from X to Y with domain D,thegraphof T is Γ(T ):= x, Dx : x D(T ) H K. {h i ∈ } ⊂ × Note that Γ(T )isasubspaceofX Y . × Definition 29.4. An operator T : X Y is closedif Γ(T )isclosedinX Y. → × Remark 29.5. It is easy to see that T is closed iff for all sequences xn D such ∈ that there exists x X and y Y such that xn x and Txn y implies that x and Tx = y. ∈ ∈ → → ∈ D Let H be a Hilbert space with inner product ( , )andnorm v := (v, v). As · · k k usual we will write H for the continuous dual of H and H for the continuous ∗ ∗ p conjugate linear functionals on H. Our convention will be that ( ,v) H∗ is linear while (v, ) H is conjugate linear for all v H. · ∈ · ∈ ∗ ∈ Lemma 29.6. Suppose that T : H K is a densely defined operator between two Hilbert spaces H and K. Then → (1) T ∗ isalwaysaclosedbutnotnecessarilydenselydefined operator. (2) If T is closable, then T¯∗ = T ∗. (3) T is closable iff T ∗ : K H is densely defined. → (4) If T is closable then T¯ = T ∗∗. Proof. Suppose vn D(T ) is a sequence such that vn 0inH and Tvn k { } ⊂ → → in K as n . Then for l D(T ∗), by passing to the limit in the equality, →∞ ∈ (Tvn,l)=(vn,T∗l)welearn(k, l)=(0,T∗l)=0. Hence if T ∗ is densely defined, this implies k = 0 and hence T is closable. This proves one direction in item 3. To prove the other direction and the remaining items of the Lemma it will be useful to express the graph of T ∗ in terms of the graph of T. We do this now. Recall that k D(T ∗)andT ∗k = h iff (k, Tx)K =(h, x)H for all x D(T ). ∈ ∈ This last condition may be written as (k, y)K (h, x)H =0forall x, y Γ(T ). − h i ∈ 534 BRUCE K. DRIVER† Let V : H K K H be the unitary map defined by V x, y = y, x . With × → × h i h− i this notation, we have k, h Γ(T ∗)iff k, h V Γ(T ), i.e. h i ∈ h i ⊥ (29.1) Γ(T ∗)=(V Γ(T ))⊥ = V (Γ(T )⊥), where the last equality is a consequence of V being unitary. As a consequence of Eq. (29.1), Γ(T ∗)isalwaysclosedandhenceT ∗ is always a closed operator, and this proves item 1. Moreover if T is closable, then Γ(T ∗)=V Γ(T )⊥ = V Γ(T )⊥ = V Γ(T¯)⊥ = Γ(T¯∗) which proves item 2. Now suppose T is closable and k (T ∗). Then ⊥ D k, 0 Γ(T ∗)⊥ = V Γ(T )⊥⊥ = V Γ(T )=V Γ(T¯), h i ∈ where T¯ denotes the closure of T. This implies that 0,k Γ(T¯). But T¯ is a well defined operator (by the assumption that T is closable)h i and∈ hence k = T¯0=0. Hencewehaveshown (T ∗)⊥ = 0 which implies (T ∗)isdenseinK. This completestheproofofitem3.D { } D 4. Now assume T is closable so that T ∗ is densely defined. Using the obvi- ous analogue of Eq. (29.1) for T ∗ we learn Γ(T ∗∗)=UΓ(T ∗)⊥ where U y, x = 1 h i x, y = V − y, x . Therefore, h− i − h i Γ(T ∗∗)=UV(Γ(T )⊥)⊥ = Γ(T )=Γ(T )=Γ(T¯) − and hence T¯ = T ∗∗. Lemma 29.7. Suppose that H and K are Hilbert spaces, T : H K is a densely → defined operator which has a densely defined adjoint T ∗. Then Nul(T ∗)=Ran(T )⊥ and Nul(T¯)=Ran(T ∗)⊥ where T¯ denotes the closure of T. Proof. Suppose that k Nul(T ∗)andh (T ), then (k, Th)=(T ∗k, h)=0. ∈ ∈ D Since h (T ) is arbitrary, this proves that Nul(T ∗) Ran(T )⊥. Now suppose ∈ D ⊂ that k Ran(T )⊥. Then 0 = (k, Th) for all h D(T ). This shows that k (T ∗) ∈ ∈ ∈ D and that T ∗k =0. The assertion Nul(T¯)=Ran(T ∗)⊥ follows by replacing T by T ∗ in the equality, Nul(T ∗) = Ran(T )⊥. Definition 29.8. Aquadraticformq on H is a dense subspace (q) H called D ⊂ the domain of q and a sesquilinear form q : (q) (q) C. (Sesquilinear means that q( ,v) is linear while q(v, ) is conjugateD linear×D on →(q) for all v (q).)The · · D ∈ D form q is symmetric if q(v, w)=q(w, v) for all v, w (q),qis positive if q(v) 0(hereq(v)=q(v, v)) for all v (q), and q is∈semi-boundedD if there ≥ ∈ D 2 exists M0 (0, ) such that q(v,v) M0 v for all v (q). ∈ ∞ ≥− k k ∈ D 29.2. Lax-Milgram Methods. For the rest of this section q will be a sesquilinear form on H and to simplify notation we will write X for (q). D Theorem 29.9 (Lax-Milgram). Let q : X X C be a sesquilinear form and suppose the following added assumptions hold.× → (1) X is equipped with a Hilbertian inner product ( , )X . (2) The form q is bounded on X, i.e. there exists a· constant· C< such that ∞ q(v,w) C v X w X for all v,w X. | | ≤ k k ·k k ∈ 2 (3) The form q is coercive, i.e. there exists >0 such that q(v,v) v X for all v X. | | ≥ k k ∈ ANALYSIS TOOLS WITH APPLICATIONS 535 Then the maps : X X and † : X X∗ defined by v := q(v, ) and L → ∗ L → L · †v := q( ,v) are linear and (respectively) conjugate linear isomorphisms of Hilbert spaces.L Moreover· 1 1 1 1 − − and ( †)− − . kL k ≤ k L k ≤ Proof. The operator is bounded because L q(v,w) (29.2) v X =sup| | C v X . kL k ∗ w=0 w X ≤ k k 6 k k Similarly † is bounded with † C. L L ≤ Let β : X X∗ denote the linear Riesz isomorphism defined by β(x)=(x, )X → 1 ° ° · for x X. Define R := β− :°X ° X so that = βR, i.e. ∈ L → L v = q(v, )=(Rv, )X for all v X. L · · ∈ Notice that R is a bounded linear mapwithoperatorboundlessthanC by Eq. (29.2). Since †v (w)=q(w, v)=(Rw, v)X =(w, R∗v)X for all v,w X, L ∈ ¯ 1 ¯ we see that¡ †¢v =(,R∗v)X , i.e. R∗ = β− †, where β(x):=(x, )X =(,x)X . Since β and βL¯ are linear· and conjugate linearL isometric isomorphisms,· to finish· the 1 1 proof it suffices to show R is invertible and that R− X − . Since k k ≤ 2 (29.3) (v, R∗v)X = (Rv, v)X = q(v,v) v , | | | | | | ≥ k kX one easily concludes that Nul(R)= 0 =Nul(R∗). By Lemma 29.7, Ran(R)= { } Nul(R∗)⊥ = 0 ⊥ = X and so we have shown R : X X is injective and has a { } → 2 dense range. From Eq. (29.3) and the Schwarz inequality, v X Rv X v X , i.e. k k ≤ k k k k (29.4) Rv X v X for all v X. k k ≥ k k ∈ This inequality proves the range of R is closed. Indeed if vn is a sequence in X { } such that Rvn w X as n then Eq. (29.4) implies → ∈ →∞ vn vm X Rvn Rvm X 0asm, n . k − k ≤ k − k → →∞ Thus v := limn vn exists in X and hence w = Rv Ran(R) and so Ran(R)= X →∞ ∈ Ran(R) = X. So R : X X is a bijective map and hence invertible. By replacing 1 → 1 v by R− v in Eq. (29.4) we learn R− is bounded with operator norm no larger 1 than − . Theorem 29.10. Let q be a bounded coercive sesquilinear form on X as in Theorem 29.9. Further assume that the inclusion map i : X H is bounded and let L and → L† be the unbounded linear operators on H defined by: (L):= v X : w X q(v, w) is H - continuous , D { ∈ ∈ → } (L†):= w X : v X q(v, w) is H - continuous D { ∈ ∈ → } and for v (L) and w (L†) define Lv H and L†w H by requiring ∈ D ∈ D ∈ ∈ q(v, )=(Lv, ) and q( ,w)=(,L†w). · · · · Then (L) and (L†) are dense subspaces of X and hence of H.
Details
-
File Typepdf
-
Upload Time-
-
Content LanguagesEnglish
-
Upload UserAnonymous/Not logged-in
-
File Pages10 Page
-
File Size-