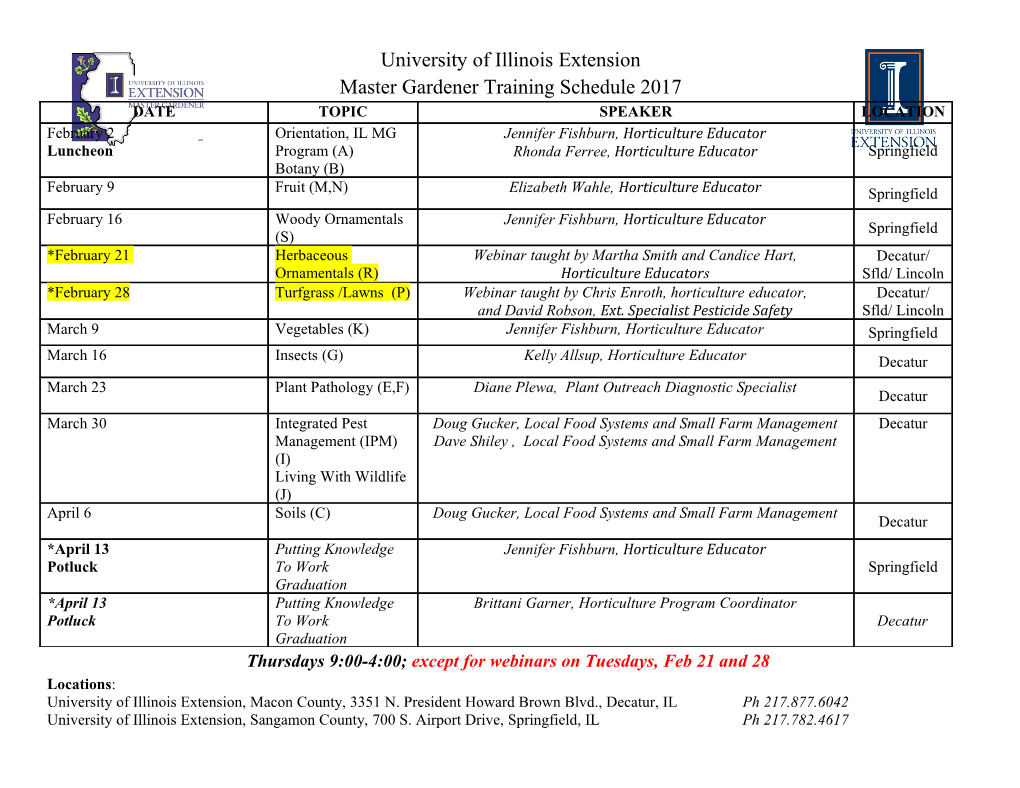
Algebraic Topology DRAFT 2013 - U.T. Contents 0 Introduction 2 1 Chain complexes 2 2 Simplicial homology 4 3 Singular homology 6 4 Chain homotopy and homotopy invariance 8 4.1 Chain homotopy . .8 4.2 Homotopy invariance . .9 5 The Snake Lemma and relative homology 10 6 The Five Lemma and excision 12 6.1 Atool ............................................. 12 6.2 Excision . 13 6.3 Locality . 14 6.4 Mayer-Vietoris sequence . 15 7 The degree of a map of spheres 15 8 Cellular homology 17 9 Cohomology and the Universal Coefficient Theorem 20 9.1 Cohomology . 20 9.2 Universal Coefficient Theorem . 21 1 0 Introduction We study topological spaces by associating algebraic objects which are invariant under homotopy (deformation). Sets: number of path components π0 Numbers: Euler characteristic χ winding number w γ Groups: fundamental group π1 ( ) Graded abelian groups: Homology H∗ Graded rings: Cohomology H∗ This course will introduce and study homology and cohomology. Why? • Nonabelian groups are difficult to work with • π1 only tells us something about the 2-skeleton of a space n • higher dimensional analogues, πn fhomotopy classes of based maps from S to the space k of interestg, are very hard to compute. Even πn S are not all know for n k. = Homology, H∗, is a refinement of the Euler characteristic and still relatively easy> to compute. References Everything contained in this course is covered in • chapters 2 and 3 of `Algebraic Topology' by Allen Hatcher, available online at http://www.math.cornell.edu/∼hatcher/ 1 Chain complexes Definition 1.1. Let C0;C1;::: be abelian groups (or vector spaces) and let @n Cn Cn−1 be homomorphisms (or linear maps). Assume that @n @n+1 0 for all n. Then ∶ → @3 @2 @1 @0 0 . ○ = = C●;@● C2 C1 C0 0 is a chain complex. Furthermore( ) define= ⋯ ÐÐ→ ÐÐ→ ÐÐ→ ÐÐÐ→ • n-chains: Cn . • n-cycles: Zn . ker @n . • n-boundaries:=Bn ( im) @n+1 Sometimes we refer to a= chain( complex) as C● for simplicity. Note 1.2. As @n @n+1 0, Bn Zn. ○ = ⊆ 2 Definition 1.3. The n-th homology of a chain complex C●;@● is . Hn C●;@● Zn Bn ker @n ( im @n)+1 : C● is called exact at Cn if Hn( 0, i.e.) = if ker @n = im( @n)+1 . (C● is) called exact if it is exact at Cn for all n 0. = ( ) = ( ) Example 1.4.≥ Let α β 0 A B C 0 be a short exact sequence of abelianÐ→ groups,ÐÐ→ thenÐÐ→ Ð→ ker α im 0 0 α is injective ker β im α A ( ) = ( ) = { } Ô⇒ ker 0 C im β β is surjective ( ) = ( ) = Thus C is isomorphic to the quotient( ) = =B A(.) Note thatÔ⇒ the group B is not determined by A and C. For example, when A Z and C Z 2Z then B could be Z or Z Z 2Z. On the otherhand, if in a short exact sequence of vector spaces~ where A, B and C are finite dimensional and all maps are linear, B is determined= by=A and~ C upto isomorphism: dim⊕ B~ dim A dim C . Definition 1.5. A chain map φ C●;@● C●; @● is a collection of homomorphisms( ) = ( ) +φn C(n ) Cn such that the following diagram commutes. ∶ ( ) → ̃ ̃ ∶ → ̃ @2 @1 @0 / C2 / C1 / C0 / 0 ⋯ φ2 φ1 φ0 / @̃2 / @̃1 / @̃0 / C2 C1 C0 0 ⋯ ̃ ̃ ̃ That is, such that φn−1 @n @n φn for all n 0. Proposition 1.6. φ C○● =C̃● induces○ a homomorphism≥ φ∗ Hn C● Hn C● for all n 0 by φ x φn x where x x Bn Zn Bn Hn C . ∗ ∶ → ̃ ● ∶ ( ) → ̃ ≥ Proof.([We]) show= [ ( that)] the map[ ] is= well-defined:+ ∈ = ( ) Let x x′ , then x x′ b for some b Bn. So φn x φn x′ b φn x′ φn b . We need φn b Bn, then φn x φn x′ . [ ] = [ ] = + ∈ ( ) = ( + ) = ( ) + ( ) ( ) ∈ ̃ [ ( )] = [ ( )] @n+1 @n Since b B , there is a b′ C such that @ b′ b. C / C / C n n+1 n+1 n+1 n n−1 Then, by commutativity, b′ / b _ ∈ ∈ ( ) = φn+1 φn φn−1 ′ φn b φn @n+1 b @̃n+1 @̃n ′ C / C / C @n+1 φn+1 b n+1 n n−1 ( ) = ( ) φn b Bm im @n 1 : ̃ ̃ ̃ = ̃ ○ + ( ) ∈ ̃ = ̃ Finally, one checks that φn x Zn: as x is a cycle, @n φn x φn−1 @n x 0. ̃ ̃ We will study three different( ) notions∈ of homology groups○ (H)n =X for○ a topological( ) = space X, which will agree when defined. ( ) 3 Simplicial Homology easy to compute and good geometric intuition Singular Homology good for theoretical work Cellular Homology better for computations and applications Through out the course we will use freely the following result from algebra. Theorem 1.7. Any finitely generated abelian group is isomorphic to r n1 n2 nk Z Z p1 Z Z p2 Z Z pk Z for some r Z≥0, k Z≥0, each⊕ pi is prime ⊕ (possibly ⊕ with ⋯ ⊕pi pj for i j) and each ni Z>0. Moreover, r; k; pi and ni are unique up to reordering. ∈ ∈ = ≠ ∈ Example 1.8. (1) Z 6Z Z 2Z Z 3Z (2) Z 9Z Z 3Z Z 3Z ≅ ⊕ ≇ ⊕ 2 Simplicial homology Definition 2.1. The standard n-simplex ∆n is n+1 t0; : : : ; tn R ti 1 and ti 0 i : Example 2.2. ( ) ∈ ∶ Q = ≥ ∀ ∆0 e2 = e1 e1 ∆2 ∆1 = = e0 e0 n k Definition 2.3. Let v0; : : : ; vn R + be such that v1 v0; : : : ; vk v0 are linearly independent. The n-simplex spanned by v0; : : : ; vn is { } ⊆ − − tivi ti 0 i and ti 1 : Its vertices are v0; : : : ; vn . LetQv0; : : : ;∶ vn ≥denote∀ theQn-simplex= spanned by v0; : : : ; vn together with the given ordering of its vertices. The ordering induces an orientation of its edges vi; vj from vi to vj. { } [ ] [ ] For any nonempty subset of the vertices, the simplex that they span is a face of v0; : : : ; vn . The vertices of this face inherit an ordering. [ ] There is a canonical homeomorphism n ∆ v0; : : : ; vn t0; : : : ; tn t0v0 tnvn: Ð→ [ ] Definition 2.4. A ∆-complex is( obtained) asz→ follows:+ ⋯ + (i) Start with an indexing set In for each n Z≥0. ∈4 n (ii) For each α In take a copy σα of the standard n-simplex. (iii) Form the disjoint union of all these simplices over all n : σn. ∈ Z≥0 n∈Z≥0 α∈In α n (iv) We require that for each n 1 -dimensional face of each n∈-simplex∐ σα there∐ is an associated n−1 n 1 -simplex σβ for some β In−1. ( − ) n (v) (Form− ) the quotient space by identifying∈ each n 1 -dimensional face of each σα with the n−1 associated simplex σβ using the canonical homeomorphism. These homeomorphisms preserve the ordering of the vertices. ( − ) Example 2.5. The torus admits the structure of a ∆-complex with one 0-simplex three 1-simplices two 2-simplices Note 2.6. The following is not a well-defined ∆-complex because the vertices of each 2-simplex are not totally ordered. Remark 2.7. Any simplicial complex is a ∆-complex, but in a ∆-complex an n-simplex may not be uniquely determined by its vertices as in Example 2.5. ∆ Definition 2.8. Let X● be a ∆-complex. The n-th chain group Cn X● of X● is the free abelian group generated by the set of n-simplices X of X . An element of C∆ X is c σn, where n ● n ● α∈In α α each cα Z and only finitely many of the cα's are non-zero. The boundary( ) homomorphism is ( ) ∑ @ C∆ X C∆ X ∈ n n ● n−1 ● i v0; : : : ; vn 1 v0;:::; v^i; : : : ; vn ∶ ( ) Ð→ i ( ) [ ] z→ Q(− ) [ ] . where v0;:::; v^i; : : : ; vn v0; : : : ; vi−1; vi+1; : : : ; vn . Example[ 2.9. ] = [ ] v2 v1 v1 1 1 @ @2 1 ⎛ ⎞ v0 v ⎛ × − ⎞ ⎛ ⎞ ⎛ + 0 ⎞ ⎜ v v ⎟ 1 × ⎜ 0 1 ⎟ = ⎜ ⎟ ⎜ ⎟ = ⎜ ⎟ ⎜ ⎟ ⎜ ⎟ ⎝ ⎠ ⎝ − ⎠ ⎝ ⎠ ⎝ × ⎠ Key Lemma 2.10. @n−1 @n 0 n Proof. ○ = ∀ i @n−1 @n v0; : : : ; vn @n−1 1 v0;:::; v^i; : : : ; vn i i j ○ ([ ]) = 1Q(−1 ) [v0 :::; v^j;:::; v^i; :] : : ; vn j<i i j−1 = Q(− ) (−1 ) [ 1 v0 :::; v^i;:::; v^j;] : : : ; vn j>i 0: + Q(− ) (− ) [ ] = 5 ∆ Definition 2.11. For n 0 the n-th simplicial homology group Hn X● is the n-th homology group of the chain complex ≥ ( ) @ @ C∆ X n+1 C∆ X n C∆ X n+1 ● n ● n−1 ● 1 1 Example 2.12. X● ⋯SÐ→S ( ) ÐÐ→ ( ) ÐÐ→ ( ) Ð→ ⋯ = ∨ / ∆ / ∆ @1 / ∆ / C2 X● C1 X● C0 X● 0 a b ⋯ v (0 ) Z ( Z ) Z( ) generated generated by v by a⊕and b @1 a v v and @1 b v v so ( ) = − ( ) H= 0 −X● ker @0 im @1 Z 0 Z H X ker @ im @ 0 1( ●) = ( 1) ( 1) = Z{ }Z= Z Z H X 0 for n 2: n( ●) = ( ) ( ) = ( ⊕ ){ } = ⊕ Example 2.13. X● torus( T) = ≥ v a v = 1 2 / ∆ / ∆ @2 / ∆ @1 / ∆ / f 2 C3 X● C2 X● C1 X● C0 X 0 b b c ⋯ ( ) ( ) ( ) ( ) g 0 Z ZZ Z Z Z 0 generated generated generated by v 0 1 by f and g by a, b, c v a v ⊕ ⊕ ⊕ @1 a @1 b @1 c v v 0 and @2 f @2 g a b c so ( ) = H0( X) =● (ker) =@0 − im= @1 Z (0) = Z ( ) = + − H X ker @ im @ im @ generated by a and b 1( ●) = ( 1) ( 2) = Z{ }Z= Z 2 Z Z H X ker @ im @ ker @ 0 generated by f g 2( ●) = ( 2) ( 3) = ( ⊕ n ⊕ ) Z( ) ≅ ⊕ H X 0 for n 3: n( ●) = ( ) ( ) = ( ){ } ≅ − Example 2.14.( ) =A simplicial≥ complex with only 0-simplices (vertices) and 1-simplices (edges) is a graph G.
Details
-
File Typepdf
-
Upload Time-
-
Content LanguagesEnglish
-
Upload UserAnonymous/Not logged-in
-
File Pages22 Page
-
File Size-