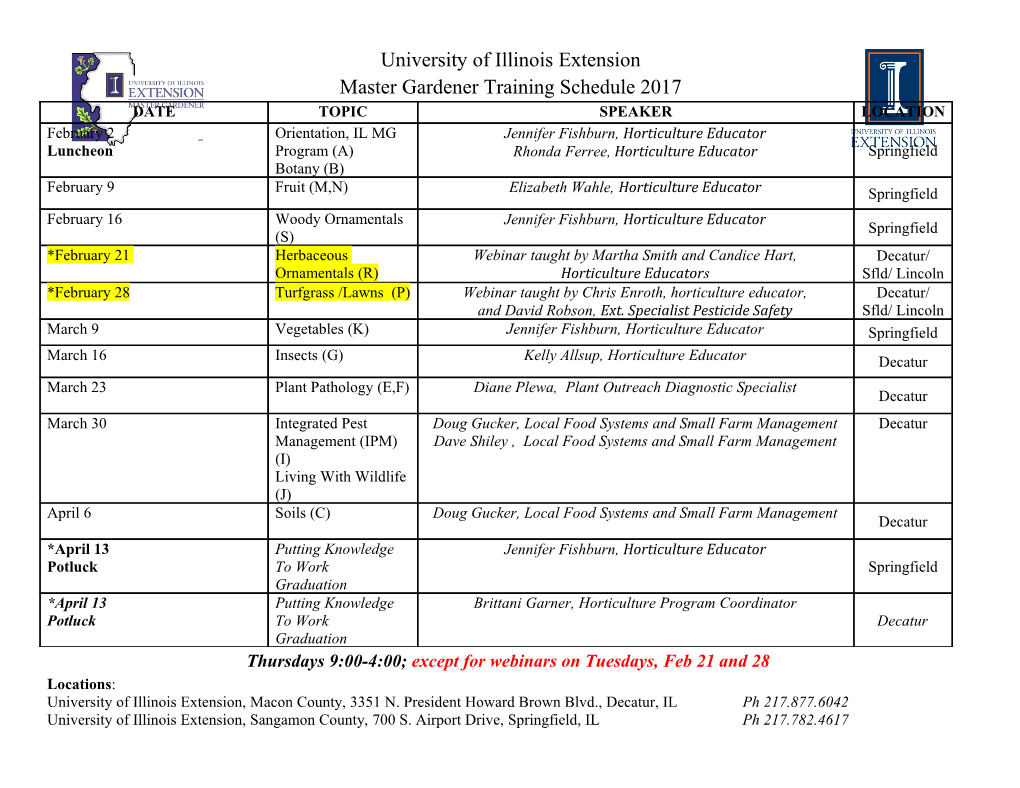
Role of surface and subsurface processes in scaling N2O emissions along riverine networks Alessandra Marzadria,1,2, Martha M. Deeb,1, Daniele Toninaa,1, Alberto Bellinc,1, and Jennifer L. Tankb,1 aCenter for Ecohydraulics Research, University of Idaho, Boise, ID 83702; bDepartment of Biological Sciences, University of Notre Dame, Notre Dame, IN 46556; and cDepartment of Civil, Environmental and Mechanical Engineering, University of Trento, Trento 38123, Italy Edited by Andrea Rinaldo, Laboratory of Ecohydrology, Ecole Polytechnique Federale Lausanne, Lausanne, Switzerland, and approved March 16, 2017 (received for review October 20, 2016) Riverine environments, such as streams and rivers, have been in the most recent Intergovernmental Panel on Climate Change reported as sources of the potent greenhouse gas nitrous oxide report are likely underestimated (6, 12–14). This uncertainty (N2O) to the atmosphere mainly via microbially mediated denitrifi- results in part from the dependence of biogeochemical reactions cation. Our limited understanding of the relative roles of the near- on complex interactions occurring at the reach scale among the surface streambed sediment (hyporheic zone), benthic, and water water column, benthic, and hyporheic zones. A key factor, which column zones in controlling N2O production precludes predic- has been elusive in previous works, is the identification of a scal- tions of N2O emissions along riverine networks. Here, we analyze ing relationship that allows us to upscale processes occurring N2O emissions from streams and rivers worldwide of different within a single reach to best quantify emissions at the riverine sizes, morphology, land cover, biomes, and climatic conditions. network scale. This scaling relationship is needed to improve cli- We show that the primary source of N2O emissions varies with mate change models that account for anthropogenic activities stream and river size and shifts from the hyporheic–benthic zone and natural processes on streams and rivers and the consequent in headwater streams to the benthic–water column zone in rivers. N2O emission from these systems. This analysis reveals that N2O production is bounded between Here, we identify and define this scaling law by linking, through two N2O emission potentials: the upper N2O emission potential a model, N2O emissions with geomorphic, hydrodynamic, and results from production within the benthic–hyporheic zone, and biogeochemical characteristics of streams and rivers and their the lower N2O emission potential reflects the production within hyporheic zones, thereby proposing a methodology to upscale SCIENCES the benthic–water column zone. By understanding the scaling reach-scale processes to riverine networks globally. Our model ENVIRONMENTAL nature of N2O production along riverine networks, our framework identifies the primary hydrodynamics and biogeochemical pro- facilitates predictions of riverine N2O emissions globally using cesses responsible for N2O emissions from streams and rivers. widely accessible chemical and hydromorphological datasets and Hydrodynamics controls the delivery of reactants to microbial thus, quantifies the effect of human activity and natural processes assemblagesanddeterminesresidencetimesforreactionstooccur on N2O production. (5, 15–17), whereas solute availability and biogeochemical activ- ity drive the conversion of solutes to gasses. This analysis shows that N O production per unit of stream/river surface area from the riverine networks j greenhouse gas j N2O j emission scaling law j 2 river network worldwide is bounded between two N O emission N2O emission potentials 2 potentials: the upper N2O emission potential is caused by produc- tion within the benthic–hyporheic interface, and the lower N2O iverine environments, such as streams and rivers, have been emission potential is caused by production within the benthic– identified as hotspots of microbially mediated denitrifica- R water column zone. These two limits emerge, because N2O emis- tion, where nitrate (NO3) is converted to both nitrogen gas sions per unit area reduce moving downstream from streams to (N2), which constitutes the majority of Earth’s atmosphere, rivers because of the systematic reduction of the hyporheic contri- and nitrous oxide (N2O), the potent greenhouse gas responsi- bution, which is only partially compensated by the increase of the ble for stratospheric ozone destruction (1). Denitrification has contribution from the water column (4, 12, 18). We assumed that been observed to occur within both bulk-oxic (2) and anoxic environments (3–5) of both benthic (i.e., sediment–water inter- face) and hyporheic (i.e., near-subsurface) zones of streams and Significance rivers. Whereas the benthic zone is the ecological region of the streambed, where both aquatic fauna and flora can be found We show that N O emissions from riverine systems depend (4, 6), the latter is the band of streambed material mainly sat- 2 on river and stream size and that the primary source of N2O urated of stream water (7). The benthic zone is at the inter- production shifts from the hyporheic and benthic zones in face between water and sediment and the upper boundary of streams to the benthic and water column in rivers. This anal- the fluvial hyporheic zone. Current understanding suggests that ysis also reveals the primary scaling factors governing river- riverine N2O production occurs predominantly in these two envi- ronments, reflecting two distinct biogeochemical transformation ine N2O emissions. Finally, it provides a predictive tool to zones (6), irrespective of system size from headwater streams quantify N2O emissions from any riverine environment world- wide, among biomes, land-use types, and climatic conditions, to rivers. The produced N2O is then exchanged with the atmo- sphere through diffusive evasion, with dynamics that depend using readily available reach-scale biogeochemical measure- on N2O concentrations of the water in relation to atmospheric ments and hydromorphological data. equilibrium (6, 8), stream hydrodynamics, temperature, and the air–water gas exchange rate (9). Although it is understood that Author contributions: A.M., M.M.D., D.T., A.B., and J.L.T. designed research; A.M., D.T., microbially mediated denitrification is responsible for a large and A.B. performed research; M.M.D. and J.L.T. analyzed data; M.M.D. and J.L.T. estab- lished synoptic sampling protocols and measurements; and A.M., M.M.D., D.T., A.B., and proportion of N2O production in riverine networks (6, 10, 11), J.L.T. wrote the paper. quantifying these emissions is challenging because of a lack of high-resolution field data and inadequate parameterization of The authors declare no conflict of interest. This article is a PNAS Direct Submission. the dominant biogeochemical processes responsible for N2O production at scales ranging from the individual reach to the 1A.M., M.M.D., D.T., A.B., and J.L.T. contributed equally to this work. river network. In addition to the uncertainty associated with pre- 2To whom correspondence should be addressed. Email: [email protected]. dictions of N2O emissions from streams and rivers, recent studies This article contains supporting information online at www.pnas.org/lookup/suppl/doi:10. suggest that global emissions from riverine networks presented 1073/pnas.1617454114/-/DCSupplemental. www.pnas.org/cgi/doi/10.1073/pnas.1617454114 PNAS Early Edition j 1 of 6 Downloaded by guest on September 24, 2021 ammonium entering via groundwater, or other routes, is quickly The work of Ocampo et al. (15) suggested interpreting NO3 nitrified, such that in-stream processes (i.e., hyporheic zone, ben- transport and transformation within a riparian zone using a thic, and water column) are the primary source of N2O emissions, Damkohler¨ number, which is the ratio of a characteristic resi- whereas direct N2O contribution, which may enter the streams dence time, with importance (28) that has been documented by from groundwater or terrestrial origin, is negligible (6). several empirical (5, 16, 17) and numerical (29, 30) investiga- We unveiled this scaling and developed our model by analyz- tions, to the characteristic time of the pertinent biogeochemi- ing available N2O emission data and hydromorphological param- cal reaction. Recent investigations also proposed this approach eters of 12 headwater streams in the Kalamazoo River (Michi- to interpret hyporheic processes (31, 32), and they adapted it gan) watershed (19, 20) and 16 headwater streams associated to quantify the hyporheic biogeochemical response at both bed- with the second Lotic Intersite Nitrogen eXperiment (LINXII) form (33) and reach (34) scales. Here, we capitalized on these Study (6, 10, 11). These data allow us to parse the relative roles advances to depict the observed scaling effect on N2O emis- of the benthic and hyporheic zones in N2O emissions and con- sions across riverine networks (Fig. 1), and we parameterized strain them between two limits: upper- and lower-bound models. the transformation efficiency of dissolved NO3 to gaseous N2O We then validated the scaling law and the identified upper- and in terms of two Damkohler¨ numbers (Materials and Methods and lower-bound models with data that we collected during synoptic SI Text). In headwater streams that are typically small and shal- sampling campaigns along the Tippecanoe River (Indiana) and low, microbially mediated denitrification occurs mainly within Manistee River (Michigan) watersheds and data available in the the benthic–hyporheic zone
Details
-
File Typepdf
-
Upload Time-
-
Content LanguagesEnglish
-
Upload UserAnonymous/Not logged-in
-
File Pages6 Page
-
File Size-