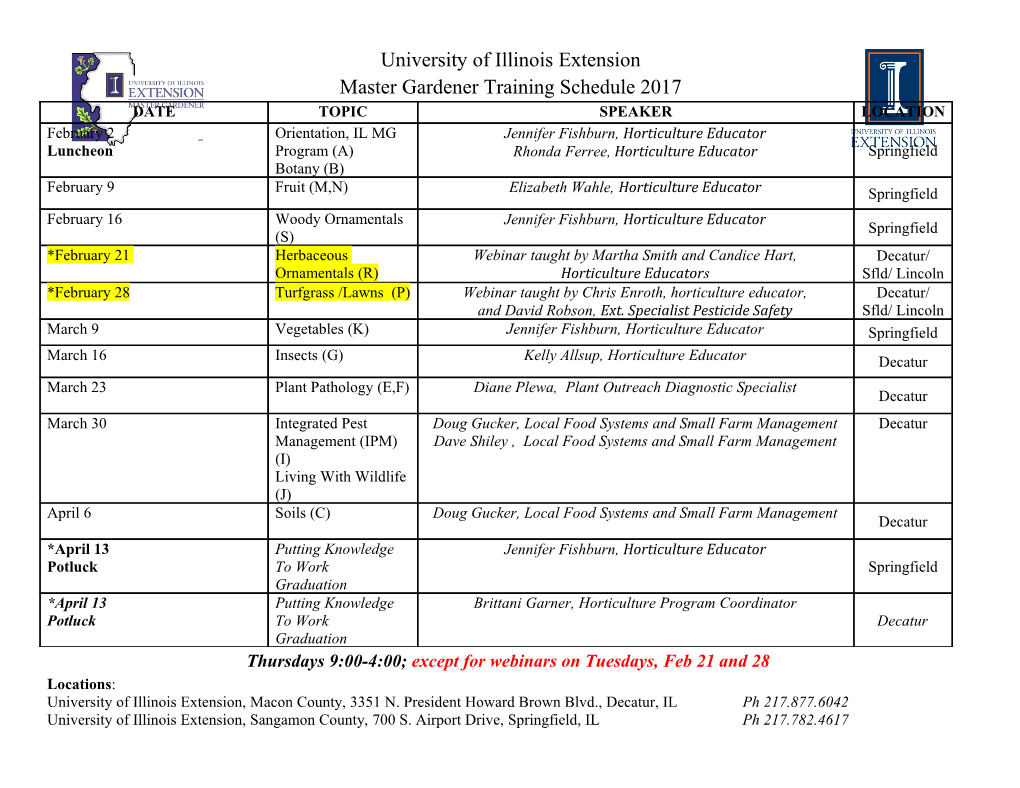
Conformational changes during the nanosecond- to-millisecond unfolding of ubiquitin Hoi Sung Chung, Munira Khalil*, Adam W. Smith, Ziad Ganim, and Andrei Tokmakoff† Department of Chemistry, Massachusetts Institute of Technology, Cambridge, MA 02139 Communicated by Robert J. Silbey, Massachusetts Institute of Technology, Cambridge, MA, November 19, 2004 (received for review September 9, 2004) Steady-state and transient conformational changes upon the ther- desirable in fast folding experiments because they have the mal unfolding of ubiquitin were investigated with nonlinear IR intrinsic time resolution to follow the fastest processes. Never- spectroscopy of the amide I vibrations. Equilibrium temperature- theless, relating these experiments to nuclear coordinates that dependent 2D IR spectroscopy reveals the unfolding of the -sheet characterize a protein structure or conformation is not trivial, of ubiquitin through the loss of cross peaks formed between particularly in the presence of disorder. transitions arising from delocalized vibrations of the -sheet. Of these probing methods, we have chosen to use vibrational Transient unfolding after a nanosecond temperature jump is mon- spectroscopy of the amide transitions of the protein backbone, itored with dispersed vibrational echo spectroscopy, a projection because it is intrinsic to all proteins and has perhaps the most of the 2D IR spectrum. Whereas the equilibrium study follows a direct connection to protein conformation. IR spectroscopy on simple two-state unfolding, the transient experiments observe amide I transitions (primarily CO stretching; 1,600–1,700 cmϪ1) complex relaxation behavior that differs for various spectral com- has been widely used for folding studies of proteins (7, 14–17) ponents and spans 6 decades in time. The transient behavior can be and peptides (9, 18–20), primarily because amide I spectra have separated into fast and slow time scales. From 100 ns to 0.5 ms, the peak positions that depend on secondary structure. The fre- spectral features associated with -sheet unfolding relax in a quency–structure correlation is a result of through-space elec- sequential, nonexponential manner, with time constants of 3 s trostatic couplings that depend on the distance and orientation and 80 s. By modeling the amide I vibrations of ubiquitin, this between the many amide I vibrations of each of the protein’s observation is explained as unfolding of the less stable strands III–V peptide units (21, 22). These couplings, which are spectroscop- of the -sheet before unfolding of the hairpin that forms part of ically significant for separations on the order of 3–8 Å, lead to the hydrophobic core. This downhill unfolding is followed by vibrational excitations that extend over large regions of the exponential barrier-crossing kinetics on a 3-ms time scale. protein and have IR transition frequencies that are sensitive to the configuration of peptide units with respect to one another protein-folding dynamics ͉ temperature jump ͉ nonlinear IR spectroscopy and hydrogen bonding to the peptide carbonyls (21, 23). Although the IR spectrum encodes information on the 3D escribing the conformational changes of proteins as they structure of the protein, traditional IR spectroscopy has been Dfold from a disordered denatured state to a compact native limited in its use as a structural probe because spectral conges- state remains an important experimental objective. Studies that tion among many overlapping features washes out the informa- examine this subject provide a molecular interpretation to the tion of interest. 13C isotope labeling, which red-shifts the labeled conceptual framework of the energy landscape picture (1–3) and amide I frequency by 35–40 cmϪ1 and is thought to localize the allow more direct comparison of experiment and simulation (4, vibration, is one approach to spectral simplification that has been 5). Viewed as a problem in molecular dynamics, characterizing used for small helical peptides (9, 18–20). To probe conforma- protein folding poses considerable challenges because it calls for tionally sensitive delocalized states and defeat spectral conges- a statistical yet structurally sensitive description of a heteroge- tion, we choose to probe protein unfolding with nonlinear IR neous ensemble in solution evolving over many decades in time. spectroscopy. Our methods use sequences of femtosecond IR Most protein-folding experiments measure kinetics: the rate pulses to interrogate vibrational couplings, dissect congested of appearance or disappearance of an experimental signature for spectra, and reveal transient structural information with pico- a particular species, typically on millisecond or longer time second time resolution. One such method is 2D IR spectroscopy, scales. These results give information on the height of energetic in which a vibrational spectrum is spread over two frequency barriers much higher than thermal energy but say little about axes, revealing couplings through the formation of cross peaks how structure changed along the path. A number of fast folding and isolating inhomogeneous broadening on the diagonal axis experiments of proteins and peptides have shown that downhill (24). As a particular strength, we have found that 2D IR folding, in which evolution is governed by energy barriers ՇkT, spectroscopy is a sensitive indicator of antiparallel -sheet can be initiated with a nanosecond temperature jump (T-jump). conformation in proteins, as a result of cross peaks that form Such experiments work in a diffusive regime that allows a freer between two characteristic IR-active vibrational modes of the exploration of available structures and provide evidence that the sheet (25–27). The shapes of these spectra are indicators of the relevant molecular time scales for folding is nanoseconds to number of strands in the sheet and its disorder. A closely related microseconds (6–9). If downhill folding can be initiated and but technically simpler nonlinear IR experiment is dispersed followed with a structure-sensitive probe, then meaningful in- vibrational echo (DVE) spectroscopy, which is related to a formation can be obtained on the underlying molecular dynam- projection of the 2D IR spectrum onto one frequency axis. As ics of folding. We report here on such an experiment, a confor- a result of this projection, DVE spectra are not as detailed as 2D mationally sensitive probing of downhill unfolding of ubiquitin IR spectra, yet they still retain a unique sensitivity to vibrational over nanosecond-to-millisecond time scales after a T-jump. The vast range of length and time scales involved in protein folding and unfolding ensures that no single experimental tech- Abbreviations: DVE, dispersed vibrational echo; FTIR, Fourier transform IR; T-jump, tem- nique can capture all relevant structural changes at high spatial perature jump. resolution in solution. Optical and IR spectroscopies such as *Present address: Lawrence Berkeley National Laboratory, Berkeley, CA 94720-8198. fluorescence-based methods (10, 11), time-resolved circular †To whom correspondence should be addressed. E-mail: [email protected]. dichroism (12), Raman (13), and IR absorption (14, 15) are © 2005 by The National Academy of Sciences of the USA 612–617 ͉ PNAS ͉ January 18, 2005 ͉ vol. 102 ͉ no. 3 www.pnas.org͞cgi͞doi͞10.1073͞pnas.0408646102 Downloaded by guest on September 23, 2021 couplings and secondary structure that is not present in con- in the supporting information, which is published on the PNAS ventional IR experiments (27). web site. We report on conformational changes during the thermal unfolding of ubiquitin studied both at equilibrium, with temper- Results ature-dependent 2D IR and DVE spectroscopy, and transiently, Equilibrium Thermal Unfolding. The conformational changes upon with DVE spectroscopy after a nanosecond T-jump. Equilibrium the thermal unfolding of ubiquitin as monitored by temperature- experiments suggest that the system obeys simple two-state dependent Fourier transform IR (FTIR), 2D IR, and DVE folding kinetics, but transient experiments reveal a more com- spectra are shown in Fig. 1. FTIR absorption spectra, shown in Ϫ plex relaxation behavior spanning 6 decades in time. From 100 Fig. 1a, are asymmetric, with a peak at 1,642 cm 1 and a shoulder Ϫ ns to 0.5 ms, we observe a nonexponential, sequential loss of two at 1,676 cm 1. Although broad and quite featureless, the asym- -sheet transitions that originate in delocalized -sheet vibra- metry is suggestive of the two-peak structure that arises from tions. On millisecond time scales, we cross into a kinetic regime, amide I vibrational couplings in antiparallel -sheets (21, 28). Ϫ characterized by a concerted 3-ms exponential unfolding. An The strong band (1,630–1,650 cm 1) has a transition moment roughly perpendicular to the -strands, and we label it Ќ, energy landscape represented in terms of native contacts be- Ϫ tween different strands of the -sheet is proposed to explain the whereas the transition moment of the weak band (Ϸ1,680 cm 1) experiments. is near parallel to the strands and is designated ʈ.Asthe temperature is raised from 58°C to 77°C, a very small decrease Methods in the peak intensity and increase in the 1,650–1,660 cmϪ1 range Nonlinear IR Spectroscopy. Detailed descriptions of the nonlinear is observed. IR experiments can be found in refs. 24, 26, and 27, including the The vibrational transitions within the congested amide I line methods of acquiring 2D IR spectra and the relationship
Details
-
File Typepdf
-
Upload Time-
-
Content LanguagesEnglish
-
Upload UserAnonymous/Not logged-in
-
File Pages6 Page
-
File Size-