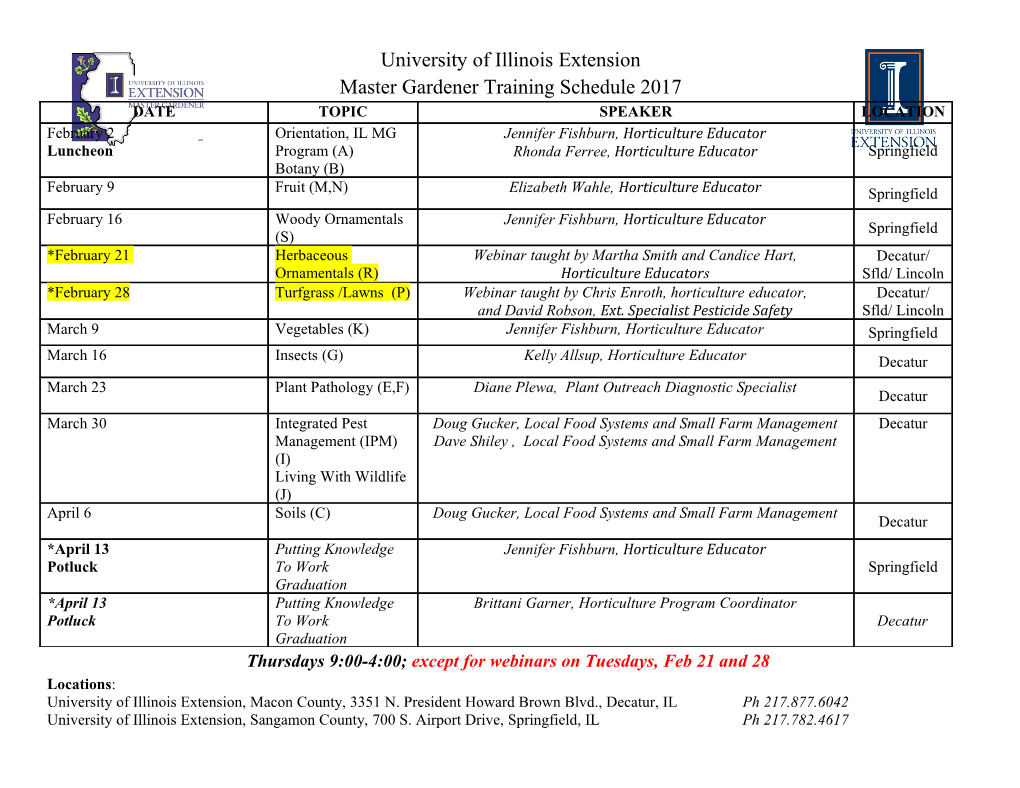
THE EFFECT OF MEMBRANES IN METHANE DEHYDROAROMATIZATION ON A BIFUNCTIONAL MO/H-ZSM-5 CATALYST IN A PACKED BED REACTOR by Benjamin Lyons Kee c Copyright by Benjamin Lyons Kee, 2016 All Rights Reserved A thesis submitted to the Faculty and the Board of Trustees of the Colorado School of Mines in partial fulfillment of the requirements for the degree of Master of Science (Mechan- ical Engineering). Golden, Colorado Date Signed: Benjamin Lyons Kee Signed: Dr. Steven DeCaluwe Thesis Advisor Signed: Dr. Canan Karakaya Thesis Advisor Golden, Colorado Date Signed: Dr. Gregory Jackson Professor and Head Department of Mechanical Engineering ii ABSTRACT Methane is becoming an increasingly attractive resource. Conversion to liquids and higher-value fuels add to the opportunities available for natural gas processes. Conventional methods for converting methane to larger hydrocarbons are through Fischer-Tropsch (F-T), which require multiple unit steps that can add inefficiencies. Methane dehydroaromatization (MDA) is a direct catalytic route to from methane to benzene. Unfortunately, the short- comings of MDA include likelihood to coke and poor yield. Conversion can be increased by removing product hydrogen via a membrane to shift the thermodynamic equilibrium towards the products. A model is developed to study the coupling of hydrogen-selective membranes with packed- bed methane dehydroaromatization (MDA) reactors using bifunctional Mo/H-ZSM-5 cata- lysts. The computational predictions are supported by previously published literature. The effect of hydrogen removal is evaluated as a function of reactor temperature, gas-hourly space velocity (GHSV), and hydrogen removal rate. Consistent with Le Chˆatelier’s principle, the results reveal that membrane integration significantly increases methane conversion. How- ever, the desired benzene yield can be diminished by the yield of undesired naphthalene and higher hydrocarbons. The benzene-to-naphthalene ratio depends strongly and nonlinearly on the membrane hydrogen removal. The results suggest that hydrogen membranes are most beneficial when the GHSV is relatively high and the catalyst temperature is relatively low. Although the single-pass benzene yield remains below 10%, the hydrogen membrane can increase benzene production rates. Hydrogen removal was also explored for MDA with steam addition. Water can preferen- tially react with naphthalene to hinder the catalyst deactivation process. Naphthalene can crack to form lighter gases such as hydrogen, ethylene, and benzene. However, condensation reactions may occur to form larger polyaromatic hydrocarbons (PAH) such as anthracene. iii Two percent steam premixed into the feed of an MDA reactor was simulated. Results suggest that water addition slightly decreases conversion, but instead improves the selectivity toward smaller hydrocarbons. Water reacts with the naphthalene to hinder PAH growth, which can lead to longer catalyst lifetime. High flow rates, low temperatures, and low hydrogen removal rates maximize non-coking benzene production. iv TABLE OF CONTENTS ABSTRACT ......................................... iii LISTOFFIGURES .....................................vii LISTOFTABLES...................................... ix LISTOFSYMBOLS......................................x ACKNOWLEDGMENTS ..................................xii DEDICATION ....................................... xiii CHAPTER1 INTRODUCTION ...............................1 1.1 Background ...................................... 1 1.1.1 MDAcatalysts ................................2 1.1.2 ChallengeswithMDA ............................4 1.1.3 MDAwithhydrogenremoval . 6 1.1.4 WateradditioninMDA ...........................7 1.2 Prior work in hydrogen selective membranes incorporated into MDA reactors . 7 1.3 Prior work in improving catalyst durability . ..........10 1.4 Overviewofpresentwork. 12 CHAPTER2 COMPUTATIONALMODEL . 13 2.1 Conservationequations. .... 14 2.2 Gas-phasetransport .............................. 14 2.3 Hydrogen-permeablemembrane . 15 2.4 Computationalmethods . 16 v 2.5 One-dimensionalmodeljustification . ........18 CHAPTER3 MDAREACTIONMECHANISM . 19 3.1 Microscopicreversibility . ...... 20 3.2 Naphthalenesteamcrackingchemistry . ...... 22 3.3 Effectofmicro-andmacroporosity . ..... 26 3.4 Model comparison against experimental data . .........29 3.5 Typicalsolution................................. 30 CHAPTER4 DRYMDARESULTS ............................ 33 4.1 Nohydrogenremoval ................................ 33 4.2 Hydrogenmembrane ................................ 37 CHAPTER5 WETMDARESULTS............................ 42 5.1 Nohydrogenremoval ................................ 42 5.2 HydrogenMembrane ................................ 46 CHAPTER 6 SUMMARY AND CONCLUSIONS . 50 6.1 Summaryofefforts ................................. 50 6.2 Keyfindings.....................................51 6.3 FutureWork.....................................52 6.4 Outlook .......................................52 REFERENCESCITED ................................... 54 vi LIST OF FIGURES Figure 1.1 Methane molecule inside H-ZSM-5 cage structure . ............3 Figure1.2 Molybdenumcarbidestructure . ........4 Figure1.3 TransientbehaviorofMDA . ......5 Figure 1.4 Membrane coupled packed-bed reactor in an annulus design .........6 Figure 1.5 Protonic-ceramic membrane coupled packed-bed reactor in an annulus design .......................................8 Figure 2.1 Illustration of the computational domain, including labeling of fluxes andreactorgeometry. ............................. 13 Figure 3.1 MDA reaction pathways proposed by Wong et al. .........20 Figure 3.2 Reaction scheme for naphthalene decomposition proposed by Devi et al. 24 Figure 3.3 Possible reaction scheme for naphthalene hydrocracking proposed by Wangetal. ...................................25 Figure 3.4 Comparison of model predictions (lines) against experimental data (markers) of Cat 1 from Sun et al. Effect of temperature on methane conversion,benzeneandaromaticyield. 27 Figure 3.5 Comparison of model predictions with the experimental data of Korobitsyna et al. The measured outlet mole fraction are shown as diamonds on the right-hand sides of the plots. .... 31 Figure 4.1 Effect of GHSV and reactor temperature on methane conversion rate for anMDAreactorwithnomembrane. 34 Figure 4.2 Effect of GHSV on benzene selectivity and methane conversion an MDA reactor with no membrane. Increase or decrease in methane conversion −1 −1 corresponds to specific GHSV values between 750-25000 mL gcat hr as itisshowninFigure4.1............................. 35 vii Figure 4.3 Effect of GHSV and reactor temperature on benzene yield for an MDA reactor with no membrane. Each data point on benzene to naphthalene −1 −1 ratio corresponds to the specific GHSV between 750-7500 mL gcat hr asitisshowninFigure4.1. 36 Figure 4.4 Effect of hydrogen removal on CH4 conversion and product yield. ◦ −1 −1 T = 700 C, GHSV = 1500 mL gcathr ...................37 Figure 4.5 Effect of hydrogen removal on (a) Methane conversion, (b) Benzene and naphthalene yields at temperatures 675 - 750 ◦C. GHSV values for all −1 −1 temperature points are 1500 mL gcat hr . ..................38 Figure 4.6 Effect of hydrogen removal on benzene formation and product −1 −1 distribution at a GHSV of 1500 mL gcat hr .................39 Figure 4.7 Effect of GHSV on C6H6 yield and formation rates in a membrane −1 −1 −1 −1 integrated MDA reactor. (a) 4500 mL gcat hr and (b) 6000 mL gcat hr . 40 Figure 4.8 Comparison of effect of GHSV on C6H6 yield and formation rate in a membrane integrated MDA reactor. Darker region indicates the desired operatingrange. ................................ 41 Figure 5.1 Effect of water addition on benzene selectivity for varying GHSV −1 −1 between 750 - 15000 mL gcat hr .......................43 Figure 5.2 Effect of water addition on methane conversion for varying GHSV −1 −1 between 750 - 15000 mL gcat hr .......................44 Figure 5.3 Effect of water addition on PAH selectivity for varying GHSV between −1 −1 750 - 15000 mL gcat hr ............................44 Figure 5.4 Effect of water addition on benzene to PAH yield ratio for varying −1 −1 GHSV between 750 - 15000 mL gcat hr ...................45 Figure 5.5 Effect of water addition on benzene formation rate . ............46 Figure 5.6 Selectivity of both benzene and PAH species for wet and dry conditions −1 −1 at a GHSV of 1500 mL gcat hr and973K ................. 47 Figure 5.7 Effect of hydrogen removal on benzene formation rate in wet conditions −1 −1 for GHSV of 1500 mL gcat hr ........................48 Figure 5.8 Effect of water addition and hydrogen removal on benzene to PAH −1 −1 species yield ratio at a GHSV of 1500 mL gcat hr .............48 viii LIST OF TABLES Table 3.1 MDA reaction mechanism on the Mo/H-ZSM-5 catalyst. ...........23 Table 3.2 Reactor and catalyst properties used in kinetic modeling of experiments reportedbySunetal. ............................. 28 Table 3.3 Comparison of model predictions against experimental data of Sun et al. at 1098 K. Effect of catalyst micro- and macroporosity on methane conversion,benzene,andaromaticyield. ..... 29 Table 3.4 Reaction conditions reported by Korobitsyna et al . .............31 Table 4.1 Major variables and parameters for dry MDA simulations .......... 33 Table 5.1 Major variables and parameters for wet MDA simulations .......... 42 ix LIST OF SYMBOLS Apparentvelocity.................................... ... v Axialcoordinate ..................................... ... z Axial
Details
-
File Typepdf
-
Upload Time-
-
Content LanguagesEnglish
-
Upload UserAnonymous/Not logged-in
-
File Pages75 Page
-
File Size-